Introduction
Cervical spondylotic radiculopathy (CSR) is the most common pattern of cervical spondylosis, which is a common degenerative disease [1]. Chronic neck and shoulder pain (CNSP) is a common clinical symptom of CSR [2]. It is estimated that over 20% of the population in Germany suffer from CNSP [3]. Many studies have investigated the underlying cerebral abnormalities caused by chronic pain, including changes in brain structure, function, chemistry, and blood flow [4-8]. A few brain imaging studies have shown that CNSP can cause changes of spontaneous neural activities [9,10].
Resting-state functional magnetic resonance imaging (rs-fMRI), widely used in chronic pain diseases [11-15], is an effective tool to evaluate the intrinsic neural activity of the human brain [16,17]. The rs-fMRI analysis methods mainly include amplitude of low-frequency fluctuation (ALFF), regional homogeneity (ReHo) [9], and functional connectivity (FC) [10]. ALFF is calculated to measure the low-frequency (0.01~0.08 Hz) spontaneous blood-oxygen-level-dependent (BOLD) fluctuations that are meaningful and are related to neural spontaneous activity [18]. The advantage of this method is that it can detect the corresponding region of impairment, which may prove to be a therapeutic target for CNSP patients in the future.
Cerebral blood flow (CBF) is closely related to brain metabolism, such as oxygen consumption, which can influence the BOLD signal [19]. Hence, ALFF does not completely explain the pathophysiological mechanism of pain. Recent studies have explored the relationship between CBF and rs-fMRI metrics [20,21]. Arterial spinlabelled (ASL) perfusion MRI permits noninvasive quantification of CBF, which can be grouped into three types: continuous labelling, pulsed labelling, and velocity selective labelling [22]. This technique has been used to explore CBF alterations in patients with cervical spondylotic myelopathy and has found increased CBF in operculum-integrated and motor control regions, and decreased CBF in sensory and motor-sensory processing regions [23]. ASL MRI therefore may provide complementary information for understanding the pathophysiology of CNSP.
To our knowledge, very few studies have simultaneously explored alterations of intrinsic brain activity and CBF in patients with CNSP. Therefore, in this study, we combined two MRI techniques, rs-fMRI and ASL, to systematically investigate alterations of ALFF and CBF in whole brain and possible relationships between ALFF and CBF. We also correlated the altered values of ALFF and CBF in CNSP patients to some clinical data. Therefore, we hypothesized that CNSP patients will present with aberrant regions of ALFF and CBF values, which might be involved in pain modulation and could become therapeutic targets to help clinicians relieve pain and improve the quality of life of CNSP patients.
Material and methods
Participants
From January 2019 to October 2019, a total of 53 righthanded individuals were recruited for this study, including 28 CNSP patients and 25 HCs. This study was approved by the Ethics Committee of the Affiliated Hospital of North Sichuan Medical College, and all the subjects voluntarily signed a informed consent form before this study.
The CNSP group met the following criteria: (1) a history of neck and shoulder pain for more than three months; (2) cervical disc herniation and nerve root compression diagnosed by conventional MRI; (3) no pain in other parts of body; and (4) did not receive any pain treatment before the study. Exclusion criteria for all subjects were as follows: (1) neck and shoulder pain caused by other reasons, such as periarthritis of shoulder; (2) structural abnormalities of the brain on conventional MRI, such as tumour, cerebral infarction, and obvious cerebral atrophy; and (3) psychiatric/neurological diseases affecting cognition.
The demographic data were recorded before the MRI scan. In order to find out some biomarkers that can predict CNSP, we also collected some clinical data, including disease duration (the time between the diagnosis and the experiment), and visual analogue scale (VAS) scores to assess the pain intensity.
Image acquisition
MRI data were acquired using a 3.0 Tesla scanner (Discovery MR750, General Electric Company) with a 32-channel head coil in our hospital. We fixed the head with a foam pad and reduced the influence of external sound with earplugs. During the scan, subjects were required to relax, close their eyes, remain awake, and think about nothing. All scan operations were performed by one skilled MRI radiologist.
The parameters of three-dimensional T1-weighted imaging (3D-T1WI) were as follows: repetition time (TR) = 5.5 ms; echo time (TE) = 1.6 ms; slices = 144; flip angle = 9°; field of view (FOV) = 240 mm × 240 mm; matrix = 256 × 256; and slice thickness = 1 mm.
Rs-fMRI was acquired using an echoplanar image sequence with parameters as follows: TR = 2000 ms; TE = 29 ms; slices = 36; flip angle = 90°; FOV = 240 mm × 240 mm; matrix = 64 × 64; and slice thickness = 3 mm.
The parameters of three-dimensional pseudo-continuous ASL (pcASL) sequence were as follows: TR = 5327 ms; TE = 10.5 ms; slices = 36; post-label delay= 2525 ms; bandwidth = 976.6 Hz; flip angle = 111°; FOV = 240 × 240 mm; matrix = 64 × 64; and slice thickness = 4 mm.
Amplitude of low-frequency fluctuation preprocessing and analysis
The rs-fMRI images were preprocessed by using the toolbox for Data Processing & Analysis of Brain Imaging (DPABI, http://rfmri.org/DPABI) based on MATLAB R2018b. The first 10 time points were removed to make the magnetic field steady and to allow the subjects adapt to the scanning environment. Then, the remaining functional images underwent slice timing to avoid the differences of slice acquisition time and were realigned to the first image for head motion correction. Next, subjects with head movements more than 1.5 mm in translation and 1.5° in rotation of any direction were excluded. Then, the corrected images were normalised to the standard Montreal Neurological Institute (MNI) space and were resampled to 3 mm cubic voxels. Next, the images were smoothed with a 4 mm full width at half maximum (FWHM) Gaussian kernel to reduce noise. After that, linear detrending and bandpass filtering (from 0.01 to 0.08 Hz) were performed to reduce the influence of low-frequency drifts and high-frequency physiological noise [24]. Finally, the white matter signal, the cerebrospinal fluid signal, the global signal, and Friston 24 head motions were removed as nuisance covariates.
The ALFF maps were calculated using the DPABI toolbox according to the ALFF method proposed by Zang et al. [25] in 2007. Then, the time series retained by the above band-pass filter were converted from time to frequency domains using the fast Fourier transform (FFT) algorithm and the power spectrum was obtained. Finally, ALFF values of each voxel were normalised by Fisher z-transformation.
Cerebral blood flow preprocessing and analysis
CBF maps were calculated automatically by a General Electric scanner. The ASL data were preprocessed by using Statistical Parametric Mapping (SPM) 12 (http://www.fil.ion.ucl.ac.uk/spm) based on MATLAB R2018b. First, the T1-weighted structural images were segmented into grey matter, white matter, and cerebrospinal fluid, which were subsequently resliced to the ASL image space. Then the CBF images were written into the MNI space using the deformation parameter derived from the prior step and were resliced into a 3 mm cubic voxel. Finally, each co-registered CBF map was spatially smoothed with a Gaussian kernel of 8 mm FWHM.
Statistical analysis
The demographics and clinical data between the two groups were compared by SPSS 23.0. Two-sample t tests were used to detect the differences in the age, education, VAS scores, and pain duration between CNSP patients and HCs. The χ2 test was used to examine the difference in sex composition between the two groups. The statistical significance level was set at a threshold of p < 0.05.
The group analysis was performed using two-sample t tests in the toolbox of DPABI software to assess the differences of ALFF and CBF between the two groups with age, gender, and education as the covariates. The results were multiple compared and corrected by AlphaSim. The mean ALFF and CBF values of the altered brain areas were extracted from each patient’s ALFF and CBF map by DPABI toolbox. Then, these abnormal values in CNSP patients were correlated to clinical data including VAS scores and pain duration by using Pearson correlation analysis in SPSS 23.0.
Results
Demographics and clinical data
The statistical results of demographics and clinical data of the two groups are listed in Table 1. There was no statistically significant difference in demographic variables between the two groups (p > 0.05).
Comparison of amplitude of low-frequency fluctuation between groups
Compared with the HCs, CNSP patients showed decreased ALFF in the left middle temporal gyrus, left precuneus, left precentral gyrus, right cingulate gyrus, right middle occipital gyrus, right middle frontal gyrus, and right postcentral gyrus, and increased ALFF in the bilateral cerebellum posterior lobe, right precuneus, left middle orbitofrontal gyrus, and left medial superior frontal gyrus (Figure 1 and Table 2).
Figure 1
The cold and warm colours, respectively, indicated that the amplitude of low-frequency fluctuation value decreased and increased in the brain regions in chronic neck and shoulder pain patients compared with healthy controls (p < 0.05, AlphaSim corrected)
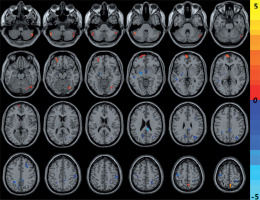
Table 2
Brain regions with amplitude of low-frequency fluctuation differences between the chronic neck and shoulder pain and healthy controls groups
Comparison of cerebral blood flow between groups
Compared with HCs, CNSP patients showed significantly decreased CBF in the left precentral gyrus and right middle frontal gyrus, and increased CBF in the bilateral superior parietal gyrus and right middle temporal gyrus (Figure 2 and Table 3).
Figure 2
The cold and warm colours, respectively, indicated that the cerebral blood flow value decreased and increased in the brain regions in chronic neck and shoulder pain patients compared with healthy controls (p < 0.05, AlphaSim corrected)
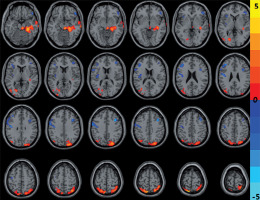
Table 3
Brain regions with cerebral blood flow differences between the chronic neck and shoulder pain and healthy controls groups
Correlations between amplitude of low-frequency fluctuation values and clinical data
As shown in Figure 3, the ALFF value of the left medial superior frontal gyrus was positively correlated with the VAS score. But there was no significant statistical relationship between the ALFF values and pain duration.
Discussion
To our knowledge, this is the first study to investigate alterations in brain function and blood flow in patients with CNSP by combining BOLD and ASL techniques. Compared to HCs, the ALFF values decreased in the left middle temporal gyrus, left precuneus, left precentral gyrus, right cingulate gyrus, right middle occipital gyrus, right middle frontal gyrus, and right postcentral gyrus, and ALFF increased in the bilateral cerebellum posterior lobe, right precuneus, left middle orbitofrontal gyrus, and left medial superior frontal gyrus. The decrease of regional CBF was mainly observed in the left precentral gyrus and right middle frontal gyrus, and the increase of regional CBF in the bilateral superior parietal gyrus and right middle temporal gyrus. These altered brain regions were pain perception and modulation, such as sensory, motor, cognitive, and emotional functions, which might improve our understanding of the central pain processing mechanisms of CNSP from the perspective of neurovascular coupling.
The default mode network
Our study found decreased ALFF in the left precuneus, right cingulate gyrus, and left middle temporal gyrus, and increased ALFF in the right precuneus. We also found increased CBF in the right middle temporal gyrus. The precuneus, cingulate gyrus, and middle temporal gyrus are parts of the default mode network (DMN) [26]. The DMN is usually active during rest and is suspended or weakened when engaged with the external environment [27,28]. The DMN is generally the primary network affected by chronic pain [29]. Baliki et al. [30] found that the DMN was modulated and reorganised by the presence of chronic pain, which reflected the maladaptive physiology of different types of chronic pain. The precuneus played a major role in a lot of advanced cognitive functions, while the cingulate cortex was involved in cognitive, affective, and motor processing functions during empathy induction [31,32]. Jiang et al. [33] demonstrated that patients with postherpetic neuralgia showed decreased connectivity in the precuneus and posterior cingulate cortex of the DMN. Although the temporal lobe is considered to be one of the brain regions of pain integration, the temporal lobe is also involved in pain perception and modulation [34,35]. Tessitore et al. [36] found that patients with migraine without aura showed decreased connectivity in the prefrontal and temporal gyrus of the DMN. In our present work, abnormal ALFF or CBF of the middle temporal gyrus, cingulate gyrus, and precuneus were detected in the brain scans. However, the altered coupling of FC and CBF between these regions within the DMN remain to be explored.
Frontal regions
In this study, the medial superior frontal gyrus and middle orbitofrontal gyrus showed increased ALFF, while the middle frontal gyrus and precentral gyrus displayed decreased ALFF. In addition, we also found that the right middle frontal gyrus of decreased ALFF was consistent with that of decreased CBF in CNSP patients. The prefrontal lobe is thought to play an important role in modulating the cortical and subcortical damaging pathways [37]. Besides pain processing, it was associated with depression and anxiety [38]. Mathew et al. [39] found that generalised anxiety disorder is associated with asymmetric increases in the N-acetylaspartate/creatine ratio, a suggested marker of neuronal viability in the prefrontal cortex. In this study, the ALFF value of the left medial superior frontal gyrus was positively correlated with VAS score. It indicated that the spontaneous nerve activity in this brain region can objectively reflect the pain intensity of CNSP patients, which may be a potential biomarker for clinical, objective evaluation of pain. The precentral gyrus is located in the frontal lobe of the cerebral cortex, which is the main somatic motor cortex [40]. A study on classical trigeminal neuralgia by Chen et al. [41] showed that the ALFF value of the right precentral gyrus was significantly higher in CTN patients when compared to HCs. Zhang et al. [21] found that elevated CBF value was observed in the right precentral gyrus of females with primary dysmenorrhea. But in this study, we found that the precentral gyrus of decreased ALFF was consistent with that of decreased CBF in CNSP patients. Therefore, our findings suggest that frontal lobe dysfunction in the processing of pain or negative emotion may be related to the generating neural mechanism of CNSP.
Parietal regions
We found decreased ALFF in the right postcentral gyrus and increased CBF in the bilateral superior parietal gyrus. The postcentral gyrus, also known as the primary somatosensory cortex (S1), is located in the lateral parietal lobe of the brain, which contributes to the processing of tactile information, as well as the suppression of painful stimuli [42,43]. Previous studies have provided inconsistent findings for this region. A chronic pain study involved increased brain connectivity to S1 in patients with rheumatoid arthritis [44]. Another study found that patients with toothaches showed significantly higher ALFF in the left postcentral gyrus [45]. We can therefore speculate that the decreased ALFF value in the postcentral gyrus indicated activation of brain activities in S1, which affected pain perception. A recent study found that patients with chronic neck pain had increased volume in the left superior parietal gyrus compared to healthy controls [46]. This finding is consistent with the higher perfusion of bilateral superior parietal gyrus in this study.
Occipital region
We observed decreased ALFF in the right middle occipital gyrus of CNSP patients. The middle occipital gyrus, located in the extrastriatum of the visual cortex, is pertaining to visual communication. A study suggested that patients with blindness had changes in the thickness of the middle occipital gyrus [47]. Another study reported that patients with discogenic low back and leg pain showed increased ALFF in the left middle occipital gyrus [16]. However, our study had inconsistent findings for this region. So, the abnormal ALFF value of the middle occipital gyri in our study may have been related to this phenomenon, but the specific mechanism needs further study.
Cerebellum
In this study, the bilateral cerebellum posterior lobe of CNSP patients displayed higher ALFF values relative to HCs. The cerebellum was part of the pain matrix and always activated in painful events in healthy humans and in patients with chronic pain [48,49]. Besides pain modulation, the cerebellum was associated with all dimensions in regards to pain, including emotion, cognition, sensory function, and motor control [50,51]. Borsook et al. [52] conducted two studies on the effects of brush and heat stimuli applied to healthy and neuropathic pain subjects, so they proposed that the cerebellum may modulate the emotional and cognitive experience that distinguishes the perception of pain from the appreciation of innocuous sensory stimulation. Our study suggested that the bilateral cerebellum posterior lobe might participate in secondary sensory processing and cognition in patients with CNSP.
Conclusions
We combined the rs-fMRI and ASL MRI techniques to detect altered ALFF and CBF values in specific brain regions of CNSP patients, which mainly involved pain modulation and perception. This provides complementary information to reveal potential pathological mechanisms of CNSP. We also found that the ALFF value of the left medial superior frontal gyrus was positively correlated with the VAS score. The ALFF signals could be an effective marker to reflect pain severity.
Limitations
However, several limitations existed in the current study. First, the number of our cases was small, and we will recruit more patients to provide more precise results in the future. Second, there is no scale to evaluate the subjects’ emotional states such as anxiety, depression, and so on, because different emotional state and severity may affect the brain functional network. Finally, we only observed the changes of ALFF and CBF in given brain regions in rs-fMRI. The altered coupling of FC and CBF should be included to reflect the neurovascular network integration in the follow-up study.