Introduction
Venous thrombosis and its complications, in particular pulmonary embolism, constitute a significant clinical problem. Incidence of venous thrombosis is estimated at 1 per 1000 per year in the general population [1], but may increase up to five per 1000 per year among subjects older than 70 years [2]. Pulmonary embolism is diagnosed in 40-60% of patients with venous thrombosis [3] and may contribute to up to 10% of deaths occurring during hospitalisation [4,5].
The principal goal of primary treatment in venous thrombosis is to prevent further enlargement of the thrombus and reduce the risk of potentially fatal pulmonary embolism [6]. Age of thrombosis is a key factor driving therapeutic decisions in patients with venous thrombosis. Early, elastic thrombi are loosely bound to the vascular wall and therefore can be easily detached and become a cause of pulmonary embolism. On the other hand, early thrombosis responds well to treatment with heparins and oral anticoagulants; furthermore, it is associated with lower risk of vascular injury during a thrombectomy procedure [7,8]. In turn, older thrombi contain a larger proportion of fibrous elements and are more tightly bound to the vascular wall; this is reflected by markedly lower risk of pulmonary embolism. In such cases, treatment, either pharmacological or surgical, is usually unnecessary or even not recommended due to risk of bleeding after administration of heparins or post-thrombectomy vascular injury [9].
In a clinical setting, age of thrombosis is estimated on the basis of symptoms, history data, results of conventional ultrasonography, and other imaging studies [10]. However, clinical presentation of venous thrombosis is highly heterogeneous, and some cases may be even asymptomatic [11-13]. Moreover, conventional compression ultrasonography with duplex scanning and colour Doppler, despite more than 95% sensitivity, specificity, and positive and negative predictive value in detection of venous thrombosis [14], is not suitable for distinguishing between acute and chronic thrombi [7,15]. All these factors contributed to growing interest in ultrasound elastography as a potential method to estimate the age of venous thrombosis.
Ultrasonographic elastography is a relatively new method of diagnostic imaging, providing information on the “hardness” of tissues independently of their acoustic impedance and vascular flows [16]. Examination is based on the assumption that each tissue has its specific elasticity; this parameter can be used to distinguish between various tissues and, most importantly, to differentiate between physiological and pathological processes [17]. Mechanical properties of tissues are evaluated based on their deformity under an applied force. The softer the tissue, the greater its deformity, and vice versa. The most commonly used type of elastography in clinical practice is real-time quasi-static elastography, so-called freehand elastography. Elastographic images of examined tissues are obtained in two stages, prior to and after controlled compression [18], processed digitally, and presented in the form of an elastogram. The latter is a colour map on which differently coloured regions correspond to tissues with various hardness. Typically, elastic tissues are presented as red areas, whereas those with intermediate and low elasticity are presented as green and blue, respectively [19]. Such an elastogram is subject to a semiquantitative analysis, i.e. comparison with a reference colour scale or determination of tissue strain ratio (SR). SR is calculated as the ratio of the deformation in the region of interest to the deformation of an adjacent reference region [18].
Available evidence suggests that dynamics of changes in the structure of venous thrombi in patients with thrombosis can be reflected on elastographic images. However, the number of previous studies dealing with the problem in question is fairly limited; apart from four [14,20-22], they dealt solely with animal or ex vivo models [23-27]. Nevertheless, these studies demonstrated that the hardness of venous thrombi increases with their age, and their elastographic parameters correspond well with the microscopic structure of vessel histopathologic specimens. However, the results of published studies are still inconclusive owing a plethora of used elastographic techniques and experimental models, as well as a relatively low statistical power. Furthermore, we still do not know whether, and based on which elastographic parameters, one can differentiate between acute venous thrombosis qualifying for primary anticoagulant therapy and older lesions, in the case of which, treatment should be aimed primarily at prevention of recurrent thrombosis and/or post-thrombotic syndrome.
Status after injection of a sclerosant, polidocanol, was shown to closely resemble venous thrombosis [28]. Sclerotherapy is an established treatment for venous insufficiency. During this procedure, injection of sclerosant contributes to chemical ablation of the affected vessel: lysis of thrombocytes and erythrocytes, and degradation of lipids and phospholipids in vascular endothelium. Changes in blood morphology and endothelial injury result in hypercoagulability, and eventually promote local thrombosis. Sclerotherapy model can be used to obtain a highly heterogeneous group of patients with venous thrombosis of known age. In this study, we applied such a model to compare elastographic parameters of material obliterating the great saphenous vein seven to 21 days after polidocanol sclerotherapy.
Material and methods
Patients
The study, conducted between October 2016 and January 2017, included 60 patients with venous insufficiency, subjected to sclerotherapy at the Centre of Phlebology in Warsaw (Poland), 45 women and 15 men (mean age 51.2 ± 14.7 years, range 27-77 years). Inclusion criteria of the study were: 1) age > 18 years, 2) written, informed consent to participate, and 3) qualification for sclerotherapy of great saphenous vein; the vein must have been 3-5 mm in diameter, fully competent, or after partial proximal phlebectomy. The list of exclusion criteria contained the following: 1) history of thrombosis in great saphenous vein, 2) presence of potential risk factors for venous thrombosis (malignancy, predisposing genetic mutation, skin lesions in area drained by the great saphenous vein), 3) anatomic factor precluding adequate visualisation of the vein, 4) evidence of recanalisation during one of the control visits, and 5) incomplete follow-up documentation.
Study protocol
The study included four visits – the first visit (sclerotherapy) followed by three control visits: 1) 7 ± 1 days, 2) 14 ± 2 days, and 3) 21 ± 2 days after the procedure.
The aim of sclerotherapy, performed independently of this study, was to obliterate great saphenous vein during the course of venous insufficiency. Qualification to treatment and the procedure itself were always carried out by the same vascular surgeon. The first stage of sclerotherapy was generation of foam, made of a sclerosant, polidocanol (Aetoxysklerol; Kreussler Pharma, Germany), mixed with respiratory gases in a 1 : 4 ratio (0.5 ml of active sclerosant per 2 ml of respiratory gases). The mixture was prepared with the aid of a 2-ml syringe, three-way adaptor, and another 5-ml syringe. The foam was generated by pushing the plunger of the larger syringe 30 times and pumping its contents to the smaller syringe. Immediately thereafter, the foam was injected to the great saphenous vein, 30 cm from the saphenofemoral junction, via a 0.45 x 16 26G x 5/8 needle. The sclerosant was administered slowly, using low pressure and under ultrasonographic control.
During each control visit, all patients were subjected to elastographic examination with the same MyLabTMTwice (Esaote SpA, Italy) ultrasonograph equipped with a linear vascular probe, type LA533. The obliterated vessel was examined in a longitudinal elastographic view (no diagnostic images were obtained in a transverse view) 30 cm from the saphenofemoral junction. To obtain the elastogram, the vein was compressed rhythmically, approximately twice per second. Correctness of the elastogram was verified against a colour pattern displayed at the side of the screen. The list of analysed parameters included total area of vessel cross-section (mm2), as well as the relative areas (%) covered by tissues the with highest, intermediate, and lowest elasticity, highlighted in on the elastograms with red, green, and blue, respectively.
Statistical analysis
Normal distribution of analysed continuous variables was verified with Shapiro-Wilk test, and their statistical characteristics were presented as arithmetic means, standard deviations, medians, and ranges. Distributions of discrete variables were presented as numbers and percentages. The significance of differences in the values of quantitative variables determined during consecutive control visits was verified with Friedman ANOVA and Wilcoxon post-hoc test. Distributions of discrete variables during consecutive visits were compared with Pearson chi-squared test and Fisher exact test. During the last stage of the analysis, we used probit regression models to estimate time to partial and complete organisation of the obliterating material. The aim of probit regression analysis is to identify the value of an explanatory variable (in this case, follow-up time) at which the study endpoint (in this case, partial or complete organisation of obliterating material) was achieved by 50% of the study subjects. All statistical calculations were carried out with Statistica 10 package (StatSoft, United States), with the threshold of statistical significance set at p ≤ 0.05.
Results
None of the study subjects showed recanalisation of the obliterating material during consecutive control visits. Statistical characteristics of elastographic findings are presented in Table 1. The area of vessel cross-section did not change significantly from visit to visit (Z = 1.405, p = 0.495). In turn, the relative area covered by tissues highlighted in red (R) decreased significantly, either between the first and the second (Z = 6.736, p < 0.001) or between the second and third control visit (Z = 6.518, p < 0.001). In contrast, the relative area covered by tissues highlighted in green (G) increased significantly between the first and the second visit (Z = 4.278, p < 0.001) and then underwent a statistically significant reduction between the second and the third visit (Z = 5.419, p < 0.001). Total relative area covered by tissues highlighted in red or green (R+G) decreased significantly both between the first and the second (Z = 6.729, p < 0.001) and between the second and the third visit (Z = 6.717, p < 0.001). Finally, the relative area covered by tissues highlighted in blue (B) underwent a statistically significant enlargement, either between the first and the second (Z = 6.725, p < 0.001) or between the second and the third control visit (Z = 6.717, p < 0.001).
Table 1
Statistical characteristics of elastographic parameters determined during consecutive control visits
Based on the relative area covered by tissues highlighted in blue (B), obliterating material was classified as: 1) elastic – for B < 33%, 2) mixed – for B ≥ 33% but < 66%, or 3) fibrous – for B ≥ 66% (Figure 1). Based on the abovementioned criteria, material obliterating the great saphenous vein during the first control visit was classified as elastic in all cases. Using the same criteria, elastographic structure of obliterating material during the second visit was classified as elastic in 36 (60.0%) patients, mixed in 23 (38.3%), and fibrous in one (1.7%). During the third visit, the structure of the obliterating material was mixed in 43 (71.7%) subjects and fibrous in 17 (28.3%). The proportion of patients in whom the structure of obliterating material was classified as mixed increased between the first and the second visit (χ2 = 30.000, p < 0.001). In turn, between the second and the third control visit, a statistically significant increase was observed in the percentage of patients with mixed or fibrous structure of obliterating material (χ2 = 56.283, p < 0.001).
Figure 1
Obliterating material whose elastographic structure was classified as (A) elastic (age 7 days – predominance of red areas), (B) mixed, fibro-elastic (age 14 days – predominance of green areas), and (C) fibrous (age 21 days – predominance of blue areas)
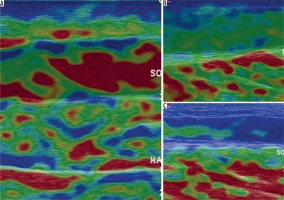
Probit regression analysis demonstrated that the mean time after which obliterating material reached mixed, fibro-elastic structure on elastographic images was 14.3 days (Figure 2). Because only 28.3% of participants reached the study endpoint (B ≥ 66%) during the third control visit, the time to complete organisation of obliterating material could be only estimated as more than 21 days (Figure 3).
Discussion
The aim of this study was to verify if elastography may provide information about the age of venous obliterating material formed after sclerotherapy procedure. The age of the obliterating material was estimated based on a relative area covered by the least elastic tissues (highlighted in blue). We chose the areas highlighted in blue because monitoring their relative size during consecutive control visits seemed to be the easiest. The relative contribution of blue areas increased significantly with the age of obliterating material, and changes in this parameter were markedly more evident than in the case of the areas highlighted in red. Although the latter parameter underwent a significant reduction over time, the dynamics of this process varied considerably from patient to patient. Importantly, similar individual variability was not observed in the case of blue areas (Table 1). Using the abovementioned approach, the character of the obliterating material at seven days post-sclerotherapy was classified as elastic in all the cases. However, the structure of the obliterating material underwent substantial changes during subsequent control visits; at 14 days post-sclerotherapy, obliterating material was classified as fibro-elastic or fibrous in 60% of the study subjects, and none of the elastograms obtained at 21 days showed the presence of an elastic obliterating material.
Our findings are consistent with the results of previous studies using elastography to estimate the age of venous thrombosis. Emelianov et al. [24] were the first to use this method to determine the age of experimentally induced thrombosis in rat inferior vena cava. They showed that the hardness of venous thrombi increased significantly with time from the induction [24]. These findings were later confirmed by Xie et al. [26], who analysed elastographic characteristics of 3- to 10-day-old thrombi induced in rat inferior vena cava. The same study demonstrated a strong positive correlation between the true and elastographically-estimated age of venous thrombi [26]. One year later the same authors [27] conducted a similar experiment, showing that the Young modulus determined elastographically in rat vena cava with experimentally induced thrombosis correlated with the same parameter measured in a mechanical ex vivo model, but solely for thrombi older than 10 days [27]. This implies that dynamics of thrombus remodelling may change over time and is not necessarily fully consistent with theoretical assumptions. This hypothesis was later confirmed by Geier et al. [25], who showed that remodelling of experimentally induced thrombi in porcine iliac veins reached its peak levels between six and 12 days post-formation [25]. Our findings are partially consistent with this observation because remodelling of obliterating material analysed in our study seemed to be markedly more intensive at 7-14 days than at 14-21 days.
We used probit logistic regression to estimate when the obliterating material had lost its elastic character and had undergone various degrees of fibrosis. The time after which the obliterating material reached mixed, fibro-elastic character (relative contribution of blue areas of at least 33% but no more than 66% of vessel cross-section) was estimated at 14.3 days (Figure 2). The follow-up period of this study (21 days) turned out to be too short to estimate the time to complete organisation of the obliterating material (Figure 3). The first attempt to estimate the age of venous thrombosis based on its elastographic parameters was undertaken by Xie et al. [26]. According to these authors, experimentally-induced thrombi in rat inferior vena cava reached complete maturation after approximately six days, which in their opinion corresponded to ca. 15 days in humans [26]. This hypothesis was partially confirmed in elastographic studies conducted by Rubin et al. [14,21]. In their first study, including two patients with deep vein thrombosis, older venous thrombi were shown to be more homogeneous and less elastic [21]. These findings were later confirmed in a markedly larger sample, including 26 patients with acute thrombosis and 28 individuals with chronic thrombosis [14]. This evidence was reflected in current therapeutic guidelines according to which invasive therapy of venous thrombosis should be undertaken no later than 14 days from its presumable onset [7,8]. Our findings correspond well with the results of previous studies. Obliterating the character of which was classified as mixed on the basis of its elastographic parameters, probably corresponded to partially mature thrombus, in the case of which radical treatment is no longer recommended. This hypothesis was further supported by the fact that the age to partial organisation of the obliterating material estimated on the basis of probit regression analysis approximated the cut-off value of 14-15 days proposed by other authors [7,8,26]. In turn, our observation that the time to complete organisation of the obliterating material was longer than 21 days is to a large extent consistent with the results published by Rubin et al. [21], according to whom thrombi older than 25 days may still undergo a partial fibrosis.
Limitations
Analysing the results of this study, one should remember that it was not conducted among patients with venous thrombosis, but in individuals subjected to sclerotherapy. Therefore, our presented findings cannot necessarily be directly transposed onto the population of patients with venous thrombosis. Nevertheless, sclerotherapy seems to be the best clinical model for venous thrombosis with known age [28]. Also, the fact that we estimated the age of the obliterating material based on the areas highlighted in blue may constitute a potential limitation of this study. Perhaps the results would be slightly different if the areas in other colour were considered as a measure of obliterating material age. Our rationale to choose blue areas, rather than those in another colour, has already been presented in this section. Another potential limitation can be too short follow-up. To guarantee comfort of our patients, we did not schedule the first control visit earlier than 6-8 days post-sclerotherapy. Therefore, we were unable to analyse remodelling of the obliterating material at the earliest stages. However, available evidence suggests that elastographically-determined remodelling of venous thrombus during the first six days is less intensive [25]. This observation was also confirmed in our study because the character of the obliterating material during the first control visit was classified as elastic in all the cases. Because we followed-up our patients for no more than 21 days, we were also unable to determine the exact time to complete organisation of the obliterating material. Nevertheless, our findings suggest that the time point at which the structure of the obliterating material changed from elastic to mixed (fibro-elastic) is more important from a clinical perspective; presumably, this time point corresponds to transition from acute to chronic thrombosis.
Even considering all the potential limitations mentioned above, the presented findings imply that ultrasound elastography with semiquantitative evaluation of the obliterating material can accurately determine the age of venous thrombi. The economic burden of venous thrombosis and its complications is huge; treatment costs of this condition in the United States amount to 15,000-25,000 USD per patient [29]. Taking into account that a large proportion of complications result from inaccurate assessment of thrombus age, routine use of an inexpensive and reproducible diagnostic instrument, such as elastography, for this purpose might produce not only have medical but also economic benefits.
Conclusions
Changes occurring with time in the structure of venous obliterating material formed during polidocanol sclerotherapy are accurately reflected by the results of ultrasound elastography. Fourteen days after its formation, the obliterating material presents on elastography as a mixed, fibro-elastic structure, presumably corresponding to chronic thrombus. However, time to complete organisation of the obliterating material is longer than 21 days.