Introduction
Multiple sclerosis (MS) is a chronic, multifocal disease of the central nervous system (CNS), which results in damage to myelin sheaths and impaired neural conduction. In most cases, MS affects young people, i.e. its onset is usually observed at between 20 and 40 years of age, and in this group of patients it is the most common non-traumatic cause of motor disability.
Dynamic development of diagnostic imaging methods, including functional magnetic resonance imaging (fMRI) and MRI volumetry, enables a better understanding of the mechanisms responsible for differences in the clinical presentation between healthy subjects and patients with MS.
In the current literature there are few reports on the functional organisation of the motor centres in MS patients [1,2]. In addition, they focus mainly on the early stages of disease and are based on single fMRI techniques. Furthermore, they are also not related to volumetric measurements of the brain and its structures, which are, without a doubt, objective indicators of organic brain damage [3,4].
The aim of this study was to assess the activity of motor cortical areas in the group of MS patients with a medium degree of motor disability, as well as the volume of brain structures, with use of task-based fMRI (t-fMRI), resting-state fMRI (rs-fMRI), and volumetric MRI studies.
Material and methods
Twenty-nine MS patients and 20 age- and gender-matched healthy volunteers were enrolled in the study. One MS patient dropped out of the study during the study period due to personal reasons, so the final analysis included 28 MS patients. MS patients were treated with immunomodulating drugs (Avonex, Betaferon, Copaxone, Rebif, Extavia). They had neither demonstrated acute relapse within 30 days prior to inclusion in the study nor shown cognitive impairment that would prevent them from comprehending commands.
The degree of motor impairment in all MS patients was assessed by a rehabilitation physician or neurologist using the Expanded Disability Status Scale (EDSS), and the averaged value was 4.65 (median value 4.5). None of the healthy volunteers included in the study had a history of neurosurgical procedures or pharmacotherapy for neurological diseases. All patients and participants were right handed.
Participants underwent a single non-contrast MRI scan using a 1.5T Siemens Avanto Tim 76 × 18 scanner, with use – T1-dependent sequence: TR = 1160 ms, TE = 2.54 ms, slice thickness 0.9 mm, 192 slices, FOV 241; t-fMRI sequence: TR = 3000 ms, TE = 50 ms, slice thickness 3 mm, 38 slices, FOV 192; and rs-fMRI sequence: TR = 2500 ms, TE = 50 ms, slice thickness 3.5 mm, 32 slices, FOV 192. To minimise movement artefacts image acquisition was first performed using the T1-weighted sequence.
The t-fMRI study was conducted using a block design, consisting of consecutive 30-second stages: task and control. During the task phase, the subjects were asked to perform alternating flexion and extension movements of the fingers. During the control stage, the subjects were asked to remain motionless with their arms placed along the torso.
In the next stage, an rs-fMRI sequence was performed to assess the brain activity during rest. The subjects had their eyes closed to be fully isolated from external stimuli. All the subjects were instructed to remain awake (conscious) and not to focus on any task during this sequence.
T-fMRI data were analysed using SPM12 software. Three regions of interest (ROI) within the motor cortex: primary motor cortex (M1), premotor cortex (PMA), and supplementary motor area (SMA) were used in the BOLD signal analysis. Each region of interest was analysed by defining a sphere with a radius of 8 mm within it, the central part of which was the point with the highest excitation, the so-called local maximum. Within these spheres, the mean values of the intensity of activity, defined as peak levels, were determined. Also, the number of excited voxels within the clusters, referred to as the cluster level, was determined.
For rs-fMRI data, the following analysis was used: non-parametric statistics (cluster – level inferences, threshold free cluster enhancement), connection threshold p < 0.05 – FWE corrected (TFCE) as well as the software: CONN toolbox (version 19b) and SPM12. The studies were exposed to 4 complex stages of analysis: defining the parameters of the whole project, “data noise reduction”, which in practice meant a qualitative analysis of the images received, individual analysis consisting of seed-to-voxel analysis, i.e. correlation analysis between the signal averaged from the selected region – seed – and all voxels from grey matter, and ROI-to-ROI. The fourth step involved a group analysis.
In addition, the volume of selected brain structures was assessed in both groups. Freesurfer image analysis software, utilising volumetric (3D), isotropic T1-weighted sequence images, was used to assess volume parameters. The total encephalic volume, and the volume of the cerebral grey and white matter were subject to volumetric analysis. The volume of the grey matter nuclei, i.e. the caudate nucleus, lentiform nucleus (both putamen and globus pallidum), thalamus, as well as the cerebellum, was also thoroughly assessed.
For quantitative data (cluster level, peak level, EDSS scale) the Shapiro-Wilk test was used to determine the normality of the distribution. Depending on whether the data were of a normal distribution, appropriate parametric or non-parametric tests were used. The hypotheses were verified using Kruskal-Wallis, post hoc Dunn’s, Mann-Whitney, Wilcoxon, or t-test, and for qualitative variables they were verified using the Fisher test. In all analyses, the test value p < 0.05 was considered significant.
Results
Activity of contralateral cortical motor areas in the multiple sclerosis group compared with the group of healthy volunteers
In all studied subjects, both in healthy volunteers and the MS group, alternating flexion and extension movements of fingers activated the contralateral area of the primary motor cortex.
The contralateral premotor cortex was activated in 27 patients from the MS group, which accounted for 96% of the studied group and in 18 healthy volunteers, which accounted for 90% of the studied group.
Activation of the contralateral supplementary motor area was observed in 14 patients from the MS group (which represented 50% of the studied group) and 12 healthy volunteers (which represented 60% of the studied group).
The mean cluster level and peak level values within the contralateral motor cortex are shown in Table 1.
Table 1
The mean cluster level and peak level values within the contralateral motor cortex
MS group | Healthy volunteers | |||
---|---|---|---|---|
Peak level | Cluster level | Peak level | Cluster level | |
Primary motor cortex | 10.26 | 71.75 | 9.58 | 71.75 |
Premotor cortex | 7.27 | 35.00 | 5.81 | 19.36 |
Supplementary motor area | 3.39 | 10.57 | 2.11 | 8.86 |
There were no statistically significant differences between the group of healthy volunteers and the MS group within contralateral areas, either in the cluster level (Figure 1) or the peak level (Figure 2) values in all analysed ROIs.
Activity of ipsilateral cortical motor areas in the multiple sclerosis group compared with the group of healthy volunteers
Statistically significant differences were observed in the frequency of activity in the ipsilateral areas of the primary and premotor cortex between patients from the MS group and the group of healthy volunteers.
In the patients from the MS group, a motor task contributed to more frequent activation of ipsilateral areas of the M1 cortex, which was observed in 11 out of 28 patients, i.e. in 39% of the studied group (p = 0.0458), while in the healthy volunteers such excitations in the same area occurred in only 2 out of 20 subjects (representing 10% of the studied group) (Figure 3).
Figure 3
Frequency of activations in the primary and premotor cortex of the ipsilateral areas in the MS group and the group of healthy volunteers. Statistically significant results are marked with an asterisk (p<0.05)
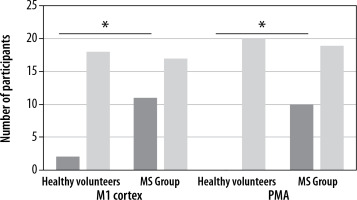
In the case of the ipsilateral premotor cortex, activation was observed in 9 out of 28 patients in the MS group (constituting 32% of the studied group, p = 0.0063), while no single healthy volunteer demonstrated excitations in the corresponding ROI.
Neither studied volunteers nor patients from the MS group demonstrated activation in the ipsilateral supplementary motor cortex.
Due to the relatively low frequency of activations found in the ipsilateral areas in both studied groups, only a qualitative analysis of these parameters was conducted.
Resting brain activity in the group of multiple sclerosis patients compared with the group of healthy volunteers
No statistically significant differences were observed in the resting brain activity between the multiple sclerosis patient group and the group of healthy volunteers based on rs-fMRI findings.
Volumetric parameters
Statistically significant differences were found between the group of healthy volunteers and MS patients in the total encephalic volume, volumes of the grey matter, cerebellum , thalamus, putamen, and globus pallidum. No statistically significant differences were detected in the volumes of the white matter and caudate nucleus (Figures 4 and 5, Tables 2 and 3).
Table 2
Pooled comparison of volumes of encephalic structures in the healthy volunteer and MS groups, part 1
Table 3
Pooled comparison of numerical values of the volume of encephalic structures in the healthy volunteers and MS groups, part 2
Figure 4
Comparison of the volume of encephalic structures in the healthy volunteer group and the MS group, part 1. Statistically significant differences are marked with an asterisk (p < 0.05)
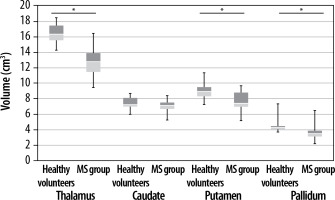
Figure 5
Comparison of the volume of encephalic structures in the healthy volunteer group and the MS group, part 2. Statistically significant differences are marked with an asterisk (p < 0.05)
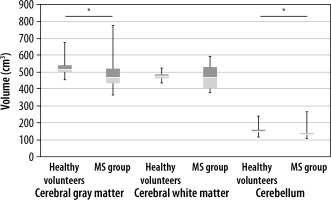
Correlation was sought between the volumes of brain structures and cluster level and peak level values within the motor cortex centres. The only correlation was revealed in the MS group between cluster level values in the contralateral premotor cortex and thalamus volume (Figure 6).
Discussion
The fMRI studies carried out so far in the group of patients with MS have confirmed the presence of functional reorganisation of the cortical centres for movement even at very early stages of the disease development, at the phase of clinically isolated syndrome (CIS), and in the absence or slight clinical impairment of motor function. However, little is known about the functioning of the above centres in patients with a medium degree of disability. So, it was very interesting to fill this research gap and to study this particular group of patients using 2 independent fMRI techniques: task and resting state fMRI. Importantly, it was done in connection with volumetric parameters, which objectively and comprehensively inform about the organic damage of the brain based on various pathomechanisms of MS development [1,3].
In the presented work, during the task phase of the t-fMRI study, the subjects were asked to perform alternating flexion and extension movements of the fingers. Within each cerebral hemisphere, 3 regions of the motor cortex, i.e. primary, premotor, and supplementary cortex, were analysed.
In both the MS group and the group of healthy volunteers, the highest activity was observed primarily in contralateral areas. In both groups the highest activity was observed in the M1 cortex, and their values were as follows: in the MS group the cluster level was 71.75 and the peak level was 10.26, whereas in the group of healthy volunteers these values were, respectively, 71.75 and 9.58. The lowest activity within contralateral areas were seen in SMA and their values were as follows: in the MS group – the cluster level was 10.57 and the peak level was 3.39, while in the group of healthy volunteers, 8.86 and 2.11, respectively.
The primary motor cortex is described as a key area involved in the generation of neural impulses and is responsible for initiation and control of movement [5]. On the other hand, the supplementary motor cortex is involved during preparation and planning of complex movements. The fact that the movement sequence in the used paradigm was characterised by a relatively low degree of complexity may explain the slight degree of activation observed in SMA in both studied groups. Such an observation also suggests that the simple movements of fingers, such as flexion and extension, did not pose a challenge, not only for healthy subjects, but, more importantly, it did not require much engagement of SMA among MS patients, who demonstrated a moderate degree of motor disability.
The authors also noted that there were no statistically significant differences in the degree of activation of contralateral areas between the group of healthy volunteers and the group of MS patients, neither in the cluster level nor in the peak level values of all determined ROIs. Such observations differ from those presented by some researchers in professional literature because in most cases they revealed more prominent activations within contralateral cortical areas in the MS group of patients and in the group of patients with CIS, which is considered the initial manifestation of multiple sclerosis – compared to healthy subjects.
Rocca et al. [6] evaluated the group of patients with CIS and compared them with an age- and gender-matched group of healthy volunteers. The analysed patients exhibited only a minimal degree of motor disability, not exceeding a value of 1 on the EDSS scale. All subjects underwent a t-fMRI study, during which they were asked to perform uncomplicated hand movements. An analysis of the cluster level parameter revealed that CIS patients showed more prominent activity in the contralateral primary and secondary somato-motor cortex compared to the control group.
Similar observations were made by Fillipi et al. [7], who assessed differences in the activity of cortical areas observed in healthy volunteers and patients with CIS while they were performing motor tasks of various degrees of difficulty. Patients with CIS demonstrated a degree of motor impairment of 0-1 on the EDSS scale. During a t-fMRI study, subjects in both groups were first asked to perform an uncomplicated motor task using their dominant hand. In the next stage, a similar task had to be performed with the non-dominant hand. In the final stage, the subjects were assigned a more complex motor task engaging not only the hand but also the lower limb. The level of activity in the contralateral primary and secondary somato-motor cortex appeared to be higher in CIS patients, who used the dominant hand while they were performing a simple motor sequence in comparison to healthy volunteers. Furthermore, it was observed that performing more complex motor tasks by patients from the CIS group not only contributed to increased activation within the contralateral primary somato-motor cortex, but also induced activations in some other regions, including the insula, thalamus, and frontal lobes, which was not observed in the control group.
Similar observations were made by Wegner et al. [8]. The authors assessed the effect of a motor task involving a sequential flexion-extension movement of all fingers of the hand on activation of cortical areas in groups of MS patients and healthy volunteers. MS patients were characterised by low levels of motor impairment, with a mean score of 2 on the EDSS scale. MS patients showed increased activation of areas located in the precentral and postcentral gyri compared to healthy volunteers, and these observations were made for both contralateral and ipsilateral areas.
In the aforementioned studies, increased activation of the contralateral cortical areas was most often interpreted as an adaptive mechanism which is responsible for compensating for neural tissue damage occurring in early stages of MS.
Results obtained in this study differ from the literature reports cited above. The lack of statistically significant differences in the activation of contralateral motor areas between MS patients and healthy volunteers may be due to various factors. On the one hand, it may be because the patients in the cited reports were either assessed at a very early stage of the disease or exhibited minimal or insignificant motor impairment. The degree of motor disability was up to 2 points on the EDSS scale, while the patients assessed in this study demonstrated more advanced motor deficits, i.e. they scored 3.9-5.4 on the Kurtzke scale. Received results might thus imply that neuroplastic changes observed during the disease have a certain limited potential, which is clearly greater in the initial phases of the disease and diminishes with the progression of motor disability. This implies that cortical functional reorganisation is not able to effectively compensate for accumulating neural tissue damage at more advanced stages of the disease.
Such interpretation of the results may be further supported by reports obtained from t-fMRI studies assessing cognitive function in MS patients. A study conducted by Hulst et al. [9] revealed reduced activation of cortical areas during a memory task in a group of patients with greater congenital impairment rather than in MS patients with preserved cognitive function.
Similar observations were made in a study by Penner et al. [10] in which the subjects were asked to focus their attention on a task. The group of MS patients demonstrated higher activation of the premotor cortex in comparison to healthy controls. However, such a relationship was not observed in MS patients with more severe cognitive impairment.
This study showed differences between MS patients and healthy volunteers in the frequency of activation of ipsilateral areas of the primary and premotor cortex. The MS group exhibited increased activation of both above-mentioned areas in comparison to healthy volunteers; in the MS group, a motor task led to activation of the M1 cortex in 11 out of 28 subjects, while in the group of healthy volunteers such excitations occurred in only 2 out of 20 subjects.
In the case of the premotor cortex, activation was found in 9 out of 28 patients in the MS group, while activations in the corresponding ROI were not observed in any single healthy volunteer.
These observations correspond to those by Reddy et al., [11] who evaluated motor function in MS patients and healthy volunteers. Participants of this study were asked to perform an uncomplicated motor task involving flexion and extension of the fingers. In the MS group of patients, the motor task resulted in increased activation of ipsilateral sensorimotor cortex areas compared to the healthy control group.
Thus, it can be concluded that the proposed motor task, being relatively simple, appeared to be more difficult for MS patients than for healthy controls. In order to properly fulfil the task, the MS patients had to engage also ipsilateral areas. Stronger activation of the CNS, including activation of ipsilateral motor cortex areas by the MS group, may have been an adaptive mechanism, triggered to better integrate motor processes, which in the course of MS are disrupted by demyelination of neural tissue.
The authors of this study did not find any statistically significant differences within the resting state networks (RSNs) between MS patients and healthy subjects, based on rs-fMRI study. The default mode network (DMN) is one of better understood functional networks. DMN is often referred to as the “inner world network” because its recruitment is associated with, among other things, analysing memories, thoughts unrelated to external stimuli, daydreaming, and conducting internal dialogue [12]. DMN has been analysed in rs-fMRI studies conducted on MS patients, and based on available literature, obtained results were often inconclusive and different depending on the assessed group.
The presented study did not reveal any differences within the DMN network or within other resting-state networks, between MS patients and healthy subjects.
These observations are consistent with findings of Roosendaal et al. [13], who analysed MS patients, CIS patients, and healthy volunteers. The authors showed higher levels of functional connectivity between areas included in DMN only in the group of patients with clinically isolated syndrome. In contrast, they did not observe similar changes in the group of MS patients or in the group of healthy individuals. Furthermore, the MS patient group demonstrated reduced functional connectivity within some RSNs, which in turn correlated with disease severity. The authors concluded that functional reorganisation within the RSN takes place primarily in early stages of the disease, as it was for CIS patients. In contrast, the ability to make reorganisation within RSN may be lost in the course of the disease.
It was also observed that MS patients exhibited increased atrophy of the whole brain in comparison to the group of healthy volunteers. Brain atrophy is a natural phenomenon and is related to the body’s natural ageing process. However, some literature reports reveal that atrophy in MS patients is more severe than in the general population [14]. The total brain tissue volume in healthy individuals is reduced each year by approximately 0.1-0.3%, while in MS patients this reduction is significantly greater, even up to 0.5-1.35%, depending on the stage of the disease and the form of pharmacotherapy. The literature also reports that patients with various forms of MS exhibit greater atrophy of grey matter, both within the cortex and subcortical areas in comparison to healthy individuals [15]. Conclusions made from most studies are consistent and reveal that the abnormal immune system response in MS not only results in the foci of demyelination but also leads to extensive changes and atrophy in the whole brain, which is a consequence of an inflammatory response, loss of axons, disruptions in the blood-brain barrier, and Wallerian degeneration [16].
Literature reports on greater brain atrophy in MS patients are consistent with the results obtained in the presented work. The mean total encephalic volume in the group of healthy volunteers was 1197 cm³, and 1150 cm³ in the group of MS patients. In addition, lower mean volumes of the grey matter were found in MS patients compared to healthy volunteers. Subcortical grey matter areas of the brain are noteworthy as MS patients, demonstrating a lower mean volume of the lenticular nuclei (the total volume of the globus pallidum and the putamen), as well as the thalamus, which is the largest centre of the mesencephalic grey matter. The thalamic nuclei demonstrate several functions, including modulation and transmission of information primarily to the cerebral cortex, including the motor cortex [17]. They play a role in the integration of sensorimotor activity, assist in the initiation of movements, and receive information about body balance. Abnormalities in the thalamus, including reduced thalamic volume, may impair the ability to perform intentional movements and predispose to hypokinesia, which is a common manifestation of MS. Its leading role in the pathomechanism of MS is highlighted by the only correlation found between its volume and the cluster level value in the contralateral premotor cortex.
Our study has certain limitations. Due to the moderate degree of mobility impairment in the MS group, it was decided not to impose a certain frequency of movements via the metronome during the task phase of the t-fMRI study. Such a situation could result in a feeling of fatigue affecting the motor centres [18]. Therefore, the frequency of movements could be different in group of patients, which could affect the results obtained. Furthermore, the study was conducted on a relatively small group. What is more, it was not fully homogeneous, even in terms of the duration of the disease and, above all, the method of treatment. It has been proven that various immunosuppressive drugs may affect the function of motor centres and their reorganisation. These limitations are common problems with studies in MS patients, but they can be minimised through extensive recruitment, which is in our current plans.
Conclusions
After a long period of development of MS leading to moderate physical disability, relatively small processes of brain plasticity are activated, involving primarily the centres of the ipsilateral hemisphere. It seems that their mobilisation, e.g. through appropriate rehabilitation, could allow motor functions to remain at the preserved level for longer.