Introduction
Dual-energy computed tomography (DECT) has been increasingly used in abdominal studies because it helps in material decomposition and also facilitates hepatic iron load differentiation, gallstones, and renal calculi. Abdominal spectral DECT has been used to analyse iodine uptake for characterisation of hepatic, renal, and adrenal lesions, assessment of bone mineral density of the lumbar spine, and to decrease beam-hardening artefacts. The post-processing of DECT data sets has also shown potential to improve image contrast and increase iodine signal intravascularly in suboptimal contrast conditions. Such techniques can also be used to reduce the volume of contrast in contrast-enhanced CT.
In the current era, because of its increased applications, DECT is routinely performed. However, the common misconception among clinicians is that DECT increases the radiation dose because the patient is scanned by dual energy levels [1]. We need convincing evidence to suggest there is no radiation dose penalty associated with DECT, so that all DECT advanced applications can be used in routine clinical practice. Previous studies have shown that second-generation DECT can be performed with no or only minimal dose penalty compared to single-energy CT (SECT) acquisition [2,3]. With the recent third-generation DSCT system, which has substantially higher X-ray tube current reserves, additional dualenergy tube voltage combinations, and a thicker tin filter for both tubes, an increase in spectral separation and further reduction in the effective radiation dose to the patient is possible.
The purpose of this study was to calculate and compare the effective radiation dose of SECT and DECT of abdomen examinations performed with third-generation dual-source CT (DSCT).
Material and methods
The study was approved by the local ethics committee and written informed consent was obtained from each participant. This was an observational study and was done during the period October 2019 to March 2020. Patients with age > 18 years, who underwent contrast-enhanced CT abdomen examinations were included in the study. Patients with absolute contraindications for iodinated contrast and scans with movement artefacts were excluded from the study.
A total of 100 patients were included and divided into 2 groups of 50 each (Group A and Group B). Groups were matched by gender (25 males and 25 females in each group) and body mass index (BMI) to allow for a direct comparison of study groups. The patients included in Group A were scanned by SECT, and Group B members were scanned by DECT.
Computed tomography technique (image acquisition and post processing)
Scans of groups A and B were performed on third-generation DSCT (Somatom Force, Siemens Healthcare Sector, Forchheim, Germany). All the patients in Group A and Group B underwent scans during the venous phase from the level of the dome of the diaphragm to the lower end of the ischial tuberosity in SECT and DECT, respectively. These patients underwent plain and arterial phases whenever there was a need according to the clinical scenario and the above-mentioned phases in both groups were performed with SECT in our institution.
Dose-optimised SECT was performed using online dose modulation (CARE Dose 4D) and automatic voltage control (Care kV, Siemens). The scan was performed with a detector collimation of 192 × 0.6 mm in craniocaudal direction and a pitch of 1 (Figures 1-3). The DECT scan was performed by using 2 different tubes voltages (100 kV and tin filtered 150 kV [Sn 150 kV]) and online dose modulation (CARE Dose 4D, Siemens). The scan was acquired with a detector collimation of 128 × 0.6 mm in craniocaudal direction and a pitch of 1 (Figures 4-6). The acquisition parameters are detailed in Table 1.
Figure 1
Axial abdominal contrast enhanced venous phase computed tomography image, acquired in single-energy mode, of a 34-year-old female with a body mass index of 22.15 kg/m2
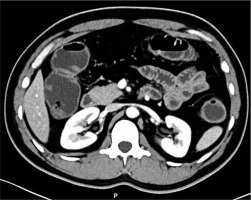
Figure 2
Coronal and sagittal reformatted abdominal contrast enhanced venous phase computed tomography images acquired in single-energy mode of the same patient
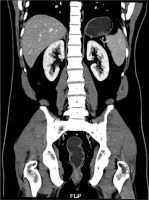
Figure 4
Axial abdominal contrast-enhanced venous phase computed tomography images acquired in dual-energy mode of a 60-year-old female with a body mass index of 22.3 kg/m2
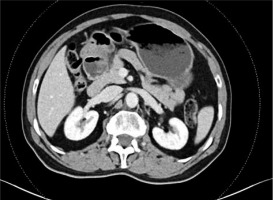
Figure 5
Coronal and sagittal reformatted abdominal contrast enhanced venous phase computed tomography images of the same patient
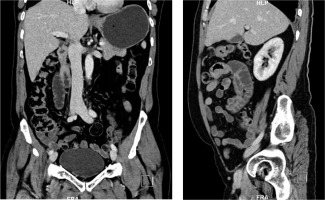
Table 1
Acquisition parameters of SECT (Group A) and DECT (Group B)
Iohexol 350 mg/ml was used as a contrast agent, and a dose of 1 ml/kg was injected at a flow rate of 4 ml/s followed by a 30 ml saline flush using an automated dualsyringe power injector through an 18 gauge IV access placed in the right median cubital vein. The timing of the scan was performed using a bolus tracking software application. The region of interest (ROI) was placed in the abdominal aorta, when HU of 200 was reached, and the scan was triggered with a delay of 4 seconds for early arterial phase, 15 seconds after bolus tracking for late arterial/early portal venous phase and 50-60 seconds delay for the venous phase.
The images in both SECT and DECT were reconstructed using a standard soft tissue reconstruction kernel (Br36) using modern iterative reconstruction technique, namely the Advanced Modeled Iterative Reconstruction (ADMIRE) algorithm, with slice thickness of 0.6 mm. Images with 3 mm thickness in axial, sagittal, and coronal planes were also generated. A single image series was created for each DECT examination using standard linear blending from the spectral datasets (weighting factor of 0.5) which corresponds to a 120 kV scan. After completion of image acquisition and reconstruction, images were transferred to a dedicated workstation (syngo.via, Siemens) for further analysis.
Radiation dose analysis
Patient protocols for each patient in the 2 groups were analysed, and the tube current, volume CT dose index (CTDIvol), dose length product (DLP), and acquisition length in cm for venous phase acquisition were recorded.
To account for variations in scan acquisition length between patients, DLP was normalised for a typical abdominal acquisition covering 40 cm. The ERD estimate was calculated for each patient by multiplying the DLP with an abdomen-specific conversion coefficient κ of 0.017 mSv/[mGy • cm] [4,5]. Previous studies have demonstrated that this conversion factor can be used for both SECT and DECT acquisition [6].
Subjective image analysis
Subjective image analysis was done by a radiologist with more than 10 years’ experience in abdominal cross-sectional imaging using the 5-point scale for anatomical details and artefacts (Table 2) in a 3 mm axial slice image dataset. This scoring criteria was developed on the basis of previous studies done by Li et al. [7] and De Ceccoa et al. [8].
Table 2
Subjective image analysis criteria based on artefacts and anatomical details
Objective image quality evaluation
The image quality was objectively evaluated using the contrast-to-noise ratio (CNR) and figure-of-merit (FOM) values. Initially CT attenuation values were measured by placing a circular ROI over the liver (ROI size, 50-100 mm2), spleen (ROI size, 50-100 mm2), portal vein (ROI size, 30-60 mm2), erector spinae muscle (ROI size, 50-100 mm2), and anterior abdominal wall subcutaneous fat (ROI size, 30-100 mm2) on 3 mm axial images of all 100 data sets. Areas with focal heterogeneity of contrast enhancement, focal calcification, and adjacent anatomical structures were excluded to facilitate homogenous measurements. The measurements were repeated 3 times to avoid data inaccuracies, and the mean values were calculated. The image noise was defined as the standard deviation of the fat.
The contrast-to-noise ratios (CNR) of the liver, spleen, and portal vein were measured by dividing the absolute difference of the attenuations within the ROI and the erector spinae muscle by the image noise. To account for differences in tube voltage settings of SECT and DECT, figure-of-merit (FOM) values were calculated [3,9]. FOM values were calculated as the ratio of CNR2 to effective radiation dose. These values were used for CNR assessment independent of ERD.
Statistical analysis
The results were collected in a Microsoft Excel file. Analyses were performed by using statistical software (SPSS version 20.0 for windows). The independent sample t test was performed. A p-value < 0.05 indicated a statistically significant difference. Bonferroni correction for multiple comparisons was applied for p-values and confidence intervals. Comparisons among the 2 groups were analysed with repeated measures analysis of variance if data were normally distributed according to the Shapiro-Wilk test. The Kruskal-Wallis 2-way analysis of variance was used if data were not normally distributed. If the effective dose was not normally distributed, the mean difference between ERD in Group A and Group B was tested through non-parametric Mann-Whitney U test.
Results
The mean age of sample Group A was 47.92 years and of Group B was 54.72 years. The age ranged between 19 and 78 years. The majority of the patients were between the age of 41 and 60 years. The mean BMI in Group A was 22.47 kg/m2 and in Group B it was 22.47 kg/m2 with a range of between 15.6 and 29.3 kg/m2. Because the patient cohorts were matched by gender and BMI, no significant differences regarding these parameters (p > 0.99) were observed, although the standard deviations varied (Table 3).
Table 3
Statistical analysis for age, height, weight, and BMI for Groups A and B
Average effective radiation dose based on DLP values normalised for 40 cm acquisition was obtained for both Group A and Group B (Table 4).
Table 4
Statistical analysis for CTDIvol, DLP, and effective dose for Groups A and B
The mean CTDI volume for Group A was 13.41 and for Group B it was 7.94. The mean DLP for Group A was 704.53 and for Group B it was 404.55. The mean ERD for Group A was 11.89 mSv and for group B it was 6.87 mSv. There was a significant difference in these values between Group A and Group B as shown by a p-value of < 0.001. The average effective radiation dose based for DLP values normalised for 40 cm acquisition was lowest in group B, with a significant difference compared to group A (p < 0.001).
Subjective score
The subjective score was analysed using a 5-point scale ranging from grade 1 to 5, where 1 was the worst and 5 was the best possible score. The mean subjective score obtained for the 2 groups (A and B) were 4.38 ± 0.52 and 4.4 ± 0.53 (mean ± SD), respectively. The p-value for individual differences of subjective score between Group A and Group B is 0.32, indicating that the difference in image quality was not statistically significant between the 2 groups (Table 5).
Objective image quality evaluation
There was no statistically significant difference in image noise between the 2 groups. The highest CNR and FOM values for liver, spleen, and portal vein were noted in the dual-energy group. However, the differences in CNR and FOM values of liver, spleen, and portal vein between both groups were not statistically significant. A detailed list of all objective image quality parameters is shown in Table 6.
Table 6
Objective image quality parameters
Discussion
With the current technological advancements, DECT has gained worldwide acceptance. In abdominal imaging, DECT examinations are mainly considered for oncological staging and follow-up, evaluation of vascular pathology, preoperative workups, and evaluation of gastrointestinal bleeding. DECT also has potential in radiation dose reduction.
The previously used first- and second-generation DSCT had certain disadvantages, such as poor spatial resolution, motion-related artefacts, and increased noise and radiation dose [10-13]. These problems were addressed in the third-generation DSCT. Although there are different software and hardware tools among the third-generation DSCT scanners for radiation dose reduction, there is much apprehension regarding the same among the clinicians [1]. We performed this study to compare the radiation dose in single- and dual-energy CT of abdomen examinations in matched patient cohort.
Evaluation of radiation dose
The results of our study show that dual-energy CT can be performed without radiation dose penalty compared to SECT with a third-generation DSCT scanner. When we evaluated a single venous phase in a matched patient cohort in third-generation DSCT using dual-energy mode, a dose reduction of 57.8% was achieved compared to single-energy CT.
The most efficient way to reduce radiation dose in CT is by adapting the scan parameters to the patient’s anatomy. Centring the patient correctly, using the right protocols, and adjusting the X-ray tube output to the patient’s size and shape help to minimize radiation exposure. Thirdgeneration DSCT has control mechanisms that automatically adjust the radiation dose to the patient’s anatomy. This technique is called CARE Dose4D, which automatically adapts the tube current to the size and shape of the patient and achieves optimal tube current modulation [14,15]. In this the tube current is first varied on the basis of a topogram, by comparing the actual patient to a “standard-sized” patient. The tube current as expected is increased for larger patients and reduced for smaller patients. The differences in attenuation in distinct body regions are also taken into account. Also, the real-time angular dose modulation measures the actual attenuation in the patient during the scan and adjusts the tube current accordingly – not only for different body regions, but also for different angles during rotation. The improved X-ray tube current capabilities of third-generation DSCT allow usage of a lower tube voltage (i.e. 100 kV) in abdominal imaging.
The next most important factor useful in reducing radiation dose in third-generation DSCT is tin filters. In third-generation DSCT, each X-ray source has a tin filter that filters out unnecessary photons for powerful low-dose scanning. It helps to achieve an optimized spectrum for dose efficiency and helps to deliver powerful low-dose scanning at the level of conventional X-ray examinations. Also, the third-generation DSCT systems are equipped with digital detectors (Stellar infinity detectors), which are more sensitive to electron influx. These detectors can measure smaller signals over a wider dynamic range, which directly enhances CT image quality especially for applications with extremely low signal levels. Such extremely low signal levels play an important role when scanning large patients and in low-dose scans, as well as in the low-kV datasets of dual-energy examinations. All the above-mentioned factors result in a significant reduction in radiation dose in third-generation dual-source CT. Our results for radiation doses with third-generation dual-energy CT (6.87 ± 4.37 mSv) averaged for 40-cm acquisitions are well below recently reported results associated with contrast-enhanced routine abdomen-pelvis CT (median of 16 mSv) [14] and are in line with the suggestions (10 mSv) from a patient education website [15].
Some of the prior studies that have investigated the radiation dose delivered by DECT compared to SECT have shown varying results. De Cecco et al. assessed second-generation DSCT protocols and compared SECT and DECT [4] and reported a small but significant increase in the radiation dose of the order 1 mSv with dual-energy CT. No significant differences in radiation dose between abdominal second-generation SECT and DECT was found in the study by Primak et al. [16]. A significant decrease in radiation dose with DECT compared to SECT in patients who underwent abdominal second-generation SECT and DECT for hepatocellular carcinoma screening was observed in a study by Purysko et al. [17]. In their study they observed a 37% reduction in overall dose with DECT compared to SECT. In our study we observed that third-generation DECT showed a significant decrease in radiation dose (57.8%) compared to SECT. Thus, dual-energy CT can be implemented in routine clinical use without negatively impacting image quality while lowering the radiation dose to the patient.
In our study, we observed the lowest radiation doses with DECT in third-generation DSCT. The mean effective radiation dose in second-generation dual-source CT in the study by Purysko et al. was 12.97 mSv [17]. However, in their study the results were not normalised for 40 cm acquisition. In a study by De Cecco et al., the mean radiation dose in second-generation dual-source CT normalised for 40 cm was 10.2 mSv [4]. In our study the mean effective radiation dose was 6.88 mSv. Our results were similar to those in the study by Wichmann et al. [3]. They concluded that with both second and third DSCT generations, abdominal DECT can be routinely performed without radiation dose penalty compared to SECT, while third-generation DSCT shows improved dose efficiency. David et al. in their study observed that the mean dose-length product (DLP) and effective dose of DECT were significantly lower than the DLP and effective dose of SECT (p < 0.05) [18]. The mean radiation dose in their study was 6.1 mSv. A reliable explanation of this reduced radiation dose could be the improved tube current capabilities in third-generation DSCT.
The other factor that plays an important role in dose reduction was modern iterative reconstruction algorithms. In our CT scan the latest generation of Siemens iterative reconstruction, called the ADMIRE algorithm, was used for the reconstruction of both SECT and DECT images. It was applied at a strength of 2 (ADMIRE 2) out of a maximum strength of 5. This level 2 strength represents a low to medium level of noise suppression due to iterative reconstruction. ADMIRE reduces image noise on CT images and maintains the spatial resolution and contrast-to-noise ratio. Some of the studies in the previous literature have mentioned that ADMIRE plays an important role in reducing radiation dose by compensating image noise with its post processing capabilities [19,20].
Further reduction in radiation does is possible in DECT scans in multiphasic examination, by utilising virtual non-contrast images and avoiding true unenhanced phase/plain scan. In a study by De Cecco et al., it was observed that by avoiding true unenhanced acquisition the radiation dose can be reduced by 32.9% [8]. Similarly, in a study by Chen et al., a decrease in radiation dose of 57% was achieved by omitting true unenhanced images in a single-phase DECT scan [21].
Evaluation of subjective criteria
The basic aim of the protocols used in this study was to generate good quality images with minimal radiation exposure. In our study we used the 5-point scoring system for assessing the subjective criteria. These scoring criteria were developed on the basis of the previous studies done by De Ceccoa et al. [8] and Li et al. [7]. All the images assessed had a score of 3 or more, indicating that they were suitable for interpretation (Figure 7). The p-value for individual differences of subjective score between Group and Group B was 0.32, indicating that the difference in image quality was not statistically significant between the 2 groups. This result is similar to the study done by Wichmann et al. [3], which showed no significant difference in image quality between single- and dual-energy scans. In a study by Tawfik et al., no significant difference in subjective image quality was observed between SECT and DECT protocols [22].
Evaluation of objective image quality
Objective image quality assessment revealed the highest values for CNR and FOM for all the assessed organs in the DECT group. In our study, the differences in the CNR and FOM values of liver, spleen, and portal vein between the SECT and DECT groups were not statistically significant. The images obtained using a third-generation dual-source scanner in SECT and DECT modes were comparable in our study. The result was similar to that from the study done by Lenga et al. [23], which showed no significant difference in objective image quality in images obtained using SECT and DECT modes in a third-generation dual-source scanner. In another study done by Lenga et al. [24], in the evaluation of chest CT, there were no statistically significant differences in CNR values of descending thoracic aorta, pulmonary trunk, and thyroid gland as well as the FOM value of the pulmonary trunk between SECT and DECT groups of a third-generation scanner.
Hence, DECT can be considered in all scenarios because it can increase both lesion detection and characterization and has the capacity to decrease artifacts and possibly contrast media dose, all while maintaining or even decreasing the radiation dose to the patient. The spectral imaging potential of DECT can be utilised routinely in abdominal DSCT.
Our study had some limitations. This study was done on the dual-source dual-energy technique. Hence, the effective radiation dose values obtained cannot be generalised to other techniques of dual-energy CT like rapid kilovolt switching, multilayer detector technique, etc., which might show different results. The study was a single institutional study with limited subjects. Further large-scale studies and meta-analysis are needed to confirm our results.
Conclusions
In third-generation dual-source dual-energy CT there is a significant reduction in the effective radiation dose to the patient compared to single-energy CT in matched cohorts. In our study, the routine abdominal examination protocols in third-generation DSCT using dual-energy mode resulted in an approximately 58% dose reduction compared to single-energy CT with similar image quality. Therefore, the quantitative imaging potential of DECT can be routinely utilised with decreased radiation dose in third-generation dual-source dual-energy CT in abdominal imaging.