Introduction
In photon-counting detector computed tomography (PCD-CT) devices the electrical signals from the CT detectors where the X-rays are detected are converted into images by software. X-rays are indirectly converted into electrical signals by flat panel detectors in 2 stages. First, the X-rays are converted into visible light through a scintillator in the upper layer [1]. In the second step, the light is absorbed by the photodiode layer and converted into electrical charges that activate pixels in the amorphous silicon layer [2,3]. The charges of each pixel are read by the reader at the bottom of the detector, and digital data is generated [1]. This data is then processed to create a digital image on the monitor [2,3]. PCDs convert X-rays into electrical signals through a semiconductor diode without an intermediary medium [1]. This review evaluates the advantages and disadvantages of PCD-CT systems.
Technologies for photon-counting detectors
Features of the PCD chips
Depending on the material, the thickness of the semiconductor diode layer might range from 1.6 to 30 mm [4,5]. A high voltage is applied to the semiconductor diode, creating a cloud that quickly separates positive and negative charges when it absorbs an incoming X-ray photon [6]. The electronic reading circuit records the electrical pulse [6]. The semiconductor detector material in the PCD immediately converts X-ray photons into electron-hole pairs upon arrival [6,7]. PCDs generate an energy pulse proportional to the energy of each photon striking the detector element [8,9].
Noise reduction and contrast-to-noise ratio improvement
An ideal PCD might generate less noise than an ideal energy integrating detector (EID) [10]. Different energy photons have different weightings in PCDs. Even in this scenario, however, it is not possible to obtain the ideal contrast-to-noise ratio (CNR). Reduced tissue contrast at high energy is the source of this. To maximise the image’s CNR, high-weight energies might be moved to lower energies. The CNR increases as a result [10].
The growing number of artifacts is a drawback of low-energy scanning [10]. Using noncontrast-material enhanced PCD-CT scanning of the human brain, the grey matter–white matter CNR increased by approximately 30% compared to the conventional CT technique [11]. Head and neck CT angiograms revealed 9% reduced picture noise compared with prior CT scans, according to Symons et al. [12].
The benefits of PCD have been noted in research conducted thus far. First, because of its high attenuation at low energies, such as calcium or iodine, ideal energy weighting can be attained [4,12]. Second, when used for low-dose imaging, PCDs outperform EIDs in terms of eliminating electronic noise. Electronic noise has very little effect on EIDs at high X-ray intensities. However, electronic noise can severely distort the image in low-dose imaging [13]. Image noise was reduced by up to 20% in human lungs using PCD-CT [14].
Resolution
The spatial resolution is related to the size of the detector element. This is approximately 1 × 1 mm² in EID systems. It is difficult to reduce the spatial resolution further due to the presence of a separator between the detector elements in the EID technique [10]. With this separator, the reflection of light photons in the scintillator is prevented [10]. In PCD, there is no such separator, and the detector element size can be reduced [15]. The detector element sizes for PCD-CT range from 0.11 × 0.11 mm² to 0.5 × 0.5 mm² [16,17]. With a smaller detector pixel size, fine details are seen in greater detail, while noise is reduced [18]. Factors affecting the quality of this detector are charge sharing and reflected X-rays, which reduce the efficiency due to fluorescent escape [16,17].
Material decomposition
Virtual monochromatic images (VMIs) can be processed to create virtual non-contrast or coloured images in the PCT technique [1]. Two or more equations are required to reconstruct an image at the desired energy level. With more energy measurements, less noisy images can be produced. The accurate measurement of the contrast material, crystals, or calculi is important for material separation [1,10,18]. Differentiation of the contrast agent was achieved by measuring 3 different materials [7]. Water, calcium, and contrast agents. Accurate measurement of these agents is possible with measurements at 3 or more energy levels [19]. EID and PCD units can be compared in terms of many factors affecting quality. Table 1 compares the 2 systems [20].
Table 1
Comparisons between conventional energy integrating detectors (EIDs) and photon-counting detectors (PCDs) [20,22,61]
Experimental studies
Significant advantages, such as a decrease in dose, an increase in spatial/geometric resolution, and a decrease in artifacts/iodine, have been observed in the PCD-CT technique [10]. Similar noise levels were observed, but a 32% dose reduction was noted in images captured with PCD compared to the EID technique [19]. Giersch et al. [21] noted that the same image can be obtained with fewer photons, resulting in a 60% dose reduction. In PCDs, more data points are obtained by using smaller detector elements [22]. Although this creates more noise for each detector, the noise level drops considerably after the reconstruction algorithms. The PCD 0.25-mm detector mode exhibited 19% less image noise in phantom, animal, and human scans in a recent study [23].
It was concluded that breast PCD-CT provides high-quality images with the potential for breast cancer screening and causes little patient discomfort [24]. Another study involving 300 women using a single-counter PCD-CT concluded that breast PCD-CT provides high-quality images and is suitable for women who do not want breast compression during mammography [25].
PCD-CT significantly improved the detection of focal liver/pancreas lesions smaller than 1 cm, tumour invasion, or liver steatosis even in the presence of iron overload [15]. Using VMIs with low-keV, PCD-CT yields significantly improved objective and subjective quality of arterial-phase oncological imaging compared with EID-CT [26]. PCD-CT imaging can detect more pancreatic cysts than conventional EID-CT [15]. It may be helpful in accurately evaluating small structures, such as enhancing mural nodules [15].
High resolution is crucial when imaging the temporal bone. Small structures cannot be diagnosed accurately at low resolution [1]. Compared with PCD-CT and high-spatial-resolution EID detectors, which had a comb filter placed in front of them, PCD-CT reduced the radiation dose, enabling clear visualisation of middle ear bones [27]. Leng et al. [18] achieved improved image quality in lung imaging with PCD-CT systems. In another study, researchers evaluated lung nodules and kidney stones. They observed that PCD-CT showed higher resolution but also higher noise [28]. Another study concluded that PCD-CT allowed for more precise lung nodule characterisation compared to conventional CT [29].
CT angiography (CTA) has replaced digital subtraction angiography (DSA) for first-line vascular imaging in routine clinical practice [30]. Fast 3D isotropic data with high-resolution acquisition is important in CTA [15,30]. PCD-CT will be useful for imaging vessels and the detection of thrombosis with optimal doses (Figures 1 and 2) [15]. Lower extremity ischaemia and below-knee artery calcification cannot be optimally evaluated using conventional CT scans due to insufficient spatial resolution. PCD-CT overcomes these issues thanks to its multi-energetic nature by reducing streak artifacts and improving signal uniformity [31,32].
Figure 1
Photon-counting computed tomography (PCCT) angiography example of the intra-cranial arteries and carotid arteries with mild atherosclerosis. The figure shows the intrapetrous internal carotid arteries (A, B) with a curved multiplanar reconstruction along the central lumen line; the bilateral bifurcation of the carotid arteries is displayed in C (right) and D (left). The scan was performed on a commercial whole-body dual-source photoncounting CT scanner (Naeotom Alpha, Siemens Healthineers, Forchheim, Germany) with 0.2 mm slice thickness, 0.1 mm reconstruction increment, and FOV 140 mm. The images are displayed with a resolution matrix of 1024 × 1024 pixels on the source axial reconstructions with a kernel filtering of Bv60 (vascular kernel medium-sharp) and with the maximum intensity of Quantum Iterative Reconstruction (QIR 4). The actual displayed resolution is 0.1 mm (100 microns)
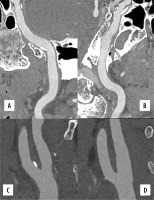
Figure 2
Photon-counting detector–computed tomography (PCD-CT) spectral angiography example of the carotid arteries with mild atherosclerosis. The figure shows spectral multi-energy axial reconstructions of carotid arteries at the level of the bifurcation; it is possible in this image to see the effect of increasing KeV directly within the standard acquisition. The scan was performed on a commercial whole-body dual-source photon-counting CT scanner (Naeotom Alpha, Siemens Healthineers, Forchheim, Germany) with 0.4 mm slice thickness, 0.2 mm reconstruction increment, and FOV 140 mm. The images are displayed with a resolution matrix of 1024 × 1024 pixels on the source axial reconstructions with a kernel filtering of Bv56 (vascular kernel medium-sharp) and with the maximum intensity of quantum iterative reconstruction (QIR 4)
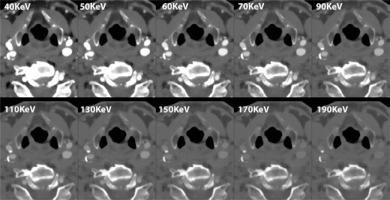
The major cause of spontaneous intracranial hypotension (SIH) is cerebrospinal fluid (CSF) leakage. Most CSF fistulas are usually 1-2 mm in diameter. Detecting small fistulas is challenging. PCD-CT can detect CSF fistulas more effectively than standard clinical imaging [10,33].
Distinguishing tumour invasion is crucial for the tumour staging of laryngeal and hypopharyngeal carcinoma [34]. However, the density of the unossified laryngeal cartilage is similar to the tumour structure in conventional CT scans, making it difficult to distinguish [34]. In such cases, PCD-CT can facilitate diagnosis [34]. In addition, PCD-CT provides better delineation of fractures, oedema, malignant involvement, and/or degenerative changes in bones and cartilage (Figure 3) [1].
Figure 3
Photon-counting computed tomography (PCCT) examples of neuro applications. The figure shows the hypothalamus (**) the hypophyseal pedicle, and the hypophysis (*; anterior hypophysis) in sagittal (A) and coronal (B) planes; in C and D a sagittal and coronal view of high-resolution of the epistropheus tooth with severe degenerative alterations. The scan was performed on a commercial whole-body dual-source photon-counting CT scanner (Naeotom Alpha, Siemens, Germany) with 0.2 mm slice thickness, 0.1 mm reconstruction increment, and FOV 140 mm. The images are displayed with a resolution matrix of 1024 × 1024 pixels on the source axial reconstructions with a kernel filtering of Bv60-80 (vascular kernel medium-sharp) and with the maximum intensity of quantum iterative reconstruction (QIR 4). The actual displayed resolution is 0.1 mm (100 microns)
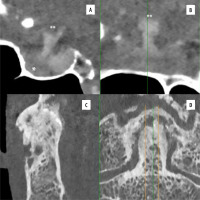
Artifacts and quality factors
The higher the quality of the image, the less distortion there is. Artifacts are one of the factors that degrade image quality. The effect of artifacts on the image is analysed in various situations and systems. CNRs of iodine were similar in 120 kV EID and 140 kV PCD scans [4]. Pourmorteza et al. [35] compared the values of 120-kV EID and 140-kV PCD based on abdominal scans. Good agreement was observed between the Hounsfield units [35].
PCD-CT eliminates beam-curing artifacts. By obtaining VMIs, the optimum CNR and iodine concentration maps can be obtained [12,36]. These data were used to distinguish intravascular contrast media from calcified plaques in the carotid arteries [31]. CT imaging may be hindered by artifacts in patients with calcified plaques, metallic implants, clips, and/or coils [37]. Metallic artifact reduction algorithms and VMIs have enhanced the image quality in PCD-CT [1,38,39]. This is especially crucial when assessing small stents made of highly attenuating materials. Recent research indicates that PCD-CT provides greater visibility and resolution of coronary lesions (Figure 4) [40,41]. Images acquired by PCD-CT showed a significant reduction in metal artifacts and an increase in dose efficiency compared with EID-CT [42,43]. Additionally, fewer streaking artifacts were observed in PCD units [44].
Figure 4
Cardiac/coronary photon-counting computed tomography (PCCT) example of a diseased coronary artery treated with an intracoronary stent. In the 2 main panels a 3D Cinematic Rendering (A), a curved multiplanar (MPR) reconstruction along the central lumen line of proximal (B; pLAD) and mid left anterior descending (B; stent). The scan was performed on a commercial whole-body dual-source photon-counting CT scanner (Naeotom Alpha, Siemens Healthineers, Forchheim, Germany) with 0.2 mm slice thickness, 0.1 mm reconstruction increment, and FOV 140 mm; the scan was performed with retrospective ECG gating with tube current modulation. In this case, the pLAD shows severe non-obstructive disease with non-calcified and calcified components down to the proximal edge of the coronary stent; the stent is perfectly patent and visible; stent struts are perfectly visible, and it is quite easy to assess the proximal calcification on the outer contour of the stent determining no issues in terms of intrastent lumen assessment. The images are displayed with a resolution matrix of 1024 × 1024 pixels on the source axial reconstructions with a kernel filtering of Bv76 (vascular kernel medium-sharp) and with the maximum intensity of quantum iterative reconstruction (QIR 4). The actual displayed resolution is 0.1 mm (100 microns)
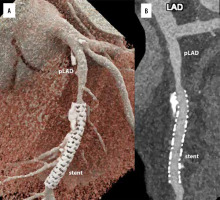
Contrast agents
Tiny vessels of hypervascular lesions, arteriovenous malformations, haemangiomas, and neoplasms can be better visualised using PCD-CT angiography [10,15]. It may also improve the CNRs, allowing for a reduction in the iodine load. The use of non-iodinated and multiple contrast agents is among the advantages that PCD-CT can provide [22]. PCD-CT allows for the removal of iodine, thereby creating virtual non-contrast images similarly to dual-energy CT [10,15].
In individuals with renal insufficiency, reducing the iodine dose is crucial. Low monochromatic image usage in dual-energy CT and reduced tube voltage in conventional CT are 2 techniques for reducing the iodine load [45-47]. PCD-CT generates monoenergetic reconstructions through energy-resolved detection and is more useful for iodine-based contrast agent detection than conventional CT detectors [48]. Iodine-specific applications might enhance the quality of coronary artery imaging (Figure 5) [31]. VMIs obtained with 40-60 keV were the best for the detection of pulmonary embolism by quantitative metrics [48]. VMIs at 60–70 keV provided better image quality in terms of vessel contrast [15].
Figure 5
Cardiac/coronary photon-counting detector–computed tomography (PCD-CT) example of mildly diseased coronary arteries. In the 4 main panels a 3D cinematic rendering (A), a curved multiplanar (MPR) reconstruction along the central lumen line of proximal left anterior descending (B; pLAD), an MPR axial/cross-sectional view of the same vessel (C) and a stretched MPR view are displayed. The scan was performed on a commercial whole-body dual-source photon-counting CT scanner (Naeotom Alpha, Siemens, Forchheim, Germany) with 0.2 mm slice thickness, 0.1 mm reconstruction increment, matrix 1024 × 1024, kernel Bv60, and FOV 140 mm; the scan was performed with retrospective ECG gating with tube current modulation. In this case, the segments of coronary arteries show a large singular eccentric calcification of the pLAD with no effect on lumen patency (no lumen diameter or area reduction) and it is displayed with a resolution matrix of 1024 × 1024 pixels on the source axial reconstructions with a kernel filtering of Bv60 (vascular kernel medium-sharp) and with maximum intensity of quantum iterative reconstruction (QIR 4). The actual displayed resolution is 0.1 mm (100 microns)
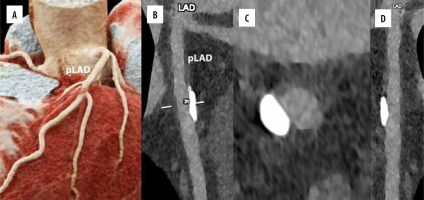
Although dual-energy CT has poor spectral separation, making it challenging to use alternative contrast agents, it does allow for various contrast agents [37,49]. In addition to iodine, barium, and gadolinium, PCD-CT can utilise high atomic number elements as contrast agents [30,37,48,49-52]. Materials that could be considered for PCD-CT include gold, platinum, xenon, bismuth, lutetium, tungsten, silver, and ytterbium [30,50-54].
It is fascinating to consider the potential use of novel contrast agents specifically designed for PCD-CT [6,30]. Gold-labelled nanoparticles have been the subject of many studies [53,54]. These metals are present in such small amounts that traditional CT scans cannot detect them. K-edge imaging and PCD-CT can be utilised for the identification and measurement of gold nanoparticles [30,53,54]. Even in the presence of additional contrast agents, such as iodine, they remain distinguishable [55].
Molecular CT imaging requires addressing safety concerns, including toxicity, stability, and patency, in addition to functionality issues, such as particle concentrations and size uniformity [6]. The distribution of particles and contrast material can be used to facilitate early cancer diagnosis [19,56]. Furthermore, the characterisation of the composition of atherosclerotic plaques can be enhanced using this method [57]. It is possible to differentiate between calcifications and iodine using dual-energy CT. It is simpler to distinguish between materials such as calcium and iodine by PCD-CT that have significant variations in their effective atomic numbers [10,18]. Dual-energy CT faces challenges in differentiating between fibrous and fatty tissues due to their significantly lower effective atomic number differences [58]. PCD-CT devices can be used to address this problem. Better material separation with enhanced spatial and temporal resolution, along with the use of targeted contrast agents, are the advantages of PCD-CT [37].
Due to PCD-CT’s ability to differentiate between different contrast agents, K-edge imaging can display various contrast materials simultaneously. As a result, it might be possible to apply multiple contrast agents simultaneously and display their distinct distribution. Targeted nanoparticles and contrast agents, such as iodine or gadolinium, can be administered simultaneously [59]. It is possible to simultaneously inject several types of nanoparticles targeting various tissue types [6]. Moreover, imaging can be performed concurrently, and multiple contrast agents can be applied at different times [53,60]. Removing the contrast agents from the images can reconstruct non-contrast material-enhanced reformatted images [61]. Multiple contrast agents can be effectively separated in vivo simultaneously using PCD-CT. Additionally, detecting bone marrow lesions or oedema is possible using virtual non-calcium images [61]. This approach reduces the radiation dose and eliminates the need for multiphase CT scans [12,14].
There are some limitations for PCD-CT systems. These limitations include increased costs, the need for larger computational, operating, or maintenance capabilities, the requirement for additional training on new acquisition/reconstruction algorithms, kernels, and technical challenges (such as charge sharing, pixel crosstalk, and pulse pile-up) [1,10]. The charge-sharing or pile-up phenomenon may lead to inaccuracies in data interpretation and reduced image quality [61]. These factors hinder the widespread use of PCD-CT scanners. In various clinical scenarios, there is a lack of data on the sensitivity and specificity of the new reconstruction algorithms, kernels, or spectral data sets [1]. Therefore, it is essential to develop and standardise high-resolution modes or scanning protocols for different body parts and specific issues [15,22].
Conclusions
PCD-CT has not yet been widely used in clinical practice, but preliminary results are promising. Among the promising features provided by PCDs are improved spatial resolution, material distinction, vessel delineation, and the ability to co-administer/apply different contrast agents. The radiation dose may be significantly reduced by PCD-CT compared to conventional EID-CT units. The fact that various quantitative maps that typically require multiple scans can now be generated with just one scan, thanks to the use of different contrast agents, indicates a reduction in dosage. Increased resolution leads to more detailed images, enabling earlier diagnosis and more accurate follow-up. Stents, coils, and vessels can be visualised more effectively with artifact reduction using PCD-CT systems and their dedicated software. PCD-CT has tremendous potential, but studies of its diagnostic ability and clinical utility for many disorders or body parts are currently limited. Further research and experience are needed before its benefits can be fully understood.