Introduction
Worldwide, the 2 main exposure sources are natural radiation and artificial sources from radiation application in medicine. The use of ionizing radiation in medical radiology has allowed physicians to see inside the human body without invasive surgery. Patients’ radiation dose exposure has exponentially increased within the last decade. It is very important to keep the dosage under control and maintain the right balance between image quality and dose, according to the ALARA principle (as low as reasonably achievable).
Medical facilities have a legal obligation to follow their patients’ doses [1,2] from medical imaging X-ray exposure, such as computed tomography (CT), interventional radiology (IR), radiography, diagnostic and screening mammography, dental radiology, and fluoroscopy.
A literature review was undertaken to identify the current recommendations for dose management and determination of the reference levels. The main assumptions of the radiation protection basic safety standards available in Europe and in the world were sought.
The currently applicable reference levels in Poland for plain radiography, CT, mammography, and fluoroscopy examinations were adopted from other countries and are presented in the Regulation of the Ministry of Health concerning the conditions of safe usage of ionizing radiation for all types of medical exposure (Dz. U. 2017 poz. 884).
International law of radiation protection
The legal regulations available in the world regarding the use of nuclear energy and ionizing radiation comprise one of the most stringent legal systems. The atomic law in individual countries is based on the recommendations of the International Atomic Energy Agency (IAEA), which was established in 1957, and the United Nations (UN) [3].
The IAEA safety standards are developed based on the findings of the United Nations Scientific Committee on the Effects of Atomic Radiation (UNSCEAR) and the recommendations of international expert bodies, in particular the International Commission for Radiological Protection (ICRP) and the International Commission on Radiation Units and Measurements (ICRU) [2,3]. Currently 172 countries are members of the IAEA [4].
The Main European regulation in the nuclear field is the European directive on Basic Safety standard 2013/59/EURATOM, which set the scene in order to protect against the dangers of exposure to ionizing radiation [5]. It obliges members of the European Union to follow the exposure of patients from medical imaging with X-rays, and it estimates population doses taking into account age and gender.
In 2014, the IAEA published Radiation Protection and Safety of Radiation Sources: International Basic Safety Standards. This publication was developed in collaboration with the European Commission, the Food and Agriculture Organization of the United Nations (FAO), the International Labour Organization (ILO), the OECD Nuclear Energy Agency (OECD/NEA), the Pan American Health Organization (PAHO), the United Nations Environment Programme (UNEP), and the World Health Organization (WHO) [6].
National law of radiation protection in Poland
In Poland, in 1957, the Central Laboratory for Radiological Protection (CLOR) was established to protect the society, environment, and people professionally exposed to the effects of ionizing radiation. The main document regulating the use of ionizing radiation in all types of activity is the Atomic Law (2021) and the executive regulations, in particular the Minister of Health regulations concerning the conditions of safe usage of ionizing radiation for all types of medical exposure (Dz. U. 2017 poz. 884). The recommendations in this legal act implements EURATOM and are in line with the general regulations of the IAEA. Supervision over compliance with the applicable legal regulations is exercised by 3 expert bodies [7]:
Nuclear regulatory bodies – National Atomic Energy Agency (mainly in radiotherapy and nuclear medicine applications);
Chief Sanitary Inspectorate (mainly in diagnostic and interventional radiology);
State Provincial Sanitary Inspectorate (mainly in diagnostic and interventional radiology).
Additionally, in Poland, the National Centre for Radiological Protection in Health Care (KCORwOZ) is implemented. The KCORwOZ was established to additionally coordinate and monitor activities related to the implementation of the radiation protection system in Poland. It has also been obliged to carry out systematic work on the implementation of the current recommendations of the European Commission in the field of radiological protection of patients in the Polish legal system. The head of the KCORwOZ is the Polish national contact person (NCP) to the UNSCEAR surveys of global radiation exposure, including medical, public, and occupational ones.
What is dose management and how is it done?
The organization and coordination of activities to review and optimize radiation doses to ensure patient safety is defined as dose management. Two main options are available for radiation exposure monitoring: manual and automated solutions.
Manual management of the collected dose data relies on writing down the dose from each performed study directly from the picture archiving and communication system (PACS), where the dose information is stored in DICOM format. However, if the diagnostic devices are not connected to the PACS, the dose should be manually written down directly from the device panel or workstation; in this case, some dose data may be missing. Subsequently, the collected data should analysed by performing some basic statistical calculation, which might be done, e.g., in MS Excel or similar software. The main disadvantages of this method are the legth of time it takes and the risk of error due to mistyping when recording the dose information [8].
For the above reasons, an automatic solution was created, which is called radiation dose tracking software (DTS) (Table 1). This digital software solution is designed to automatically collect and analyse the patient’s radiation exposure data from medical X-rays and nuclear medicine imaging devices. It supports continuous data collection from multiple devices, across multiple modalities, irrespective of the equipment vendor, and it allows preconfigured analysis, customizable alerts, and automatic reporting. DTS enables healthcare professionals to optimally monitor the radiation exposure, evaluate their practices, and make improvements so that the imaging studies provide the greatest benefit and least risk to the patient [9].
Table 1
Summary of chosen radiation dose tracking software [9]
* Additional actions are required to implement this system in a medical facility, CE mark is the medical device manufacturer’s certificate. CT – computed tomography, DR – digital radiography, DXA – dual-energy X-ray absorptiome-try, MG –mammography, NM – nuclear medicine, RF – radio fluoroscopy, XA – angiography
The advantage of DTS is the ability to set alerts for exceeding the dose for a specific type of examination. The system automatically informs about exceeding the dose; it allows users to react quickly and check what caused this unexpected event, which helps in the optimization of imaging procedures and potentially reduces the probability of the stochastic effects. The alert values can be configured by the user for each anatomic region. There are some recommended values of alerts, for example for CT, which are presented in Table 2.
Table 2
Alert values for computed tomography examinations recommended by the American Association of Physicists in Medicine [10]
The CT dose index value (volume) or DLP is compared to the alert value at each anatomic position after an examination, and when this value is exceeded, an alert will be triggered on the DTS panel; it allows users, e.g. physicists, to react quickly and optimize the examination protocol.
Quantities used for dose management for different modalities
It is very important to understand and know the dose of radiation application in medicine, which varies by each different imaging modality.
The dosimetric quantities of dose area product (DAP) and entrance surface dose (ESD) are used for projection radiography. The DAP is also used for angiography and diagnostic and interventional fluoroscopy [11-19].
For CT, 3 quantities are used: the computed tomography dose index (volume) (CTDIvol), dose length product (DLP), and size-specific dose estimate (SSDE) [11,16].
The average glandular dose (AGD) and incident air kerma (Ka,I ) are used for mammography.
In dental medicine, ionizing radiation is used for dental intraoral and dental panoramic imaging modalities. The dosimetric quantities of incident air kerma (IAK) and DAP are used. For cone-beam CT (CBCT), incident air kerma at the patient entrance reference point (CAK), CTDIvol, DLP, and DAP can be used depending on the availability of the quantity [14,18,19].
For nuclear medicine, the administered activity (amount of radioactive material), or preferably the administered activity per unit of body weight, is used [11].
Table 3 shows the quantities, symbols, and units for setting diagnostic reference levels (chapter 5) recommended by the ICRP.
Table 3
Dosimetry quantities used in different modalities of medical imaging [11]
[i] DAP – dose area product, KAP and PKA – air kerma area product, ESD – entrance surface dose, ESAK and Ka,e – entrance-surface air kerma, CAK and Ka,r – incident air kerma at the patient entrance reference point, CTDIvol – computed tomography dose index (volume), DLP – dose length product, SSDE – size-specific dose estimate, AGD – average glandular dose, DG and MGD – mean glandular dose, IAK and Ka,i – incident air kerma
Definitions
The dose area product (DAP) or kerma area product (KAP, PKA) is the product of dose and beam area (Gy cm2) and is measured using an ionization chamber placed between patient and the X-ray tube [12].
The entrance surface dose (ESD) or entrance surface air kerma (Ka,e, ESAK) is defined as the absorbed dose to air at the point of intersection of the entrance surface of the patient with the X-ray beam axis (mGy) [13].
The incident air kerma at the patient entrance reference point (CAK, Ka,r) is the air kerma at a point in space located at a fixed distance from the focal spot, which is accumulated from a whole X-ray procedure expressed in mGy [14].
The computed tomography dose index (volume) (CTDIvol) (measured in mGy) is the average absorbed dose at a point within the scan volume for a particular procedure for a standardized phantom with a 16 or 32 cm diameter [15].
The dose length product (DLP) is related to the CTDIvol. It is the parameter used as the average dose absorbed by the patient in a CT scan of length L along the z-axis. It is usually measured in mGy cm [16].
However, the CTDIvol and DLP values are calculated from a mathematical phantom that does not consider patient size.
The size-specific dose estimate (SSDE), measured in mGy, is a method of estimating the CT radiation dose, which takes a patient’s size into account. It is the CTDIvol adjusted for patient equivalent thickness based on a set of standard coefficients [17].
The average glandular dose (AGD) or mean glandular dose (DG, MGD) is an estimate of the glandular tissues of a breast to the average absorbed dose during mammography. It is measured in mGy [18].
The incident air kerma (IAK, Ka,I), measured in mGy, is the air kerma from the incident beam on the central X-ray beam axis at focal spot-to-skin distance without backscatter. The incident air kerma can be calculated from the X-ray tube output, where the output is measured using a calibrated ionizing chamber [19].
Comparison of radiation risk from radiological procedures and imaging modalities
To estimate total body radiation exposure, the effective dose (ED) is used. It is defined as the sum of the equivalent dose to the organs multiplied by tissue weighting factors, representing the specific sensitivity of organs and tissues to specific radiation types. It is measured in mSv. The ED may be used to compare doses from different imaging modalities (Figure 1). It is expressed by the following formula [20]:
where wT is the tissue weighting factor for tissue or organ T , HT is the equivalent doses to the tissues or organs, wR is the radiation weighting factor, and DT,R is the mean absorbed dose from radiation R in a tissue or organ T.
Figure 1 shows how to calculate the ED. First, determine the absorbed doses of individual tissues and organs exposed. The equivalent dose is obtained by multiplying the absorbed doses of individual tissues and organs by their respective radiation weighting factors (WR) for considering the types of radiation [21].
Second, multiply the equivalent dose by the respective tissue weighting factors (WT) to consider differences in sensitivity among organs. Then the products are summed. The ED can be expressed as the weighted average of the equivalent doses of all organs and tissues.
The radiation weighting factors depend on the types of radiation, reflecting the destructive biological effectiveness of different radiation types. The greatest biological effects are caused by α-particles, heavy ions, fission fragments, and neutrons with energy of about 100 keV – 2 MeV.
Photons are a type of radiation that is used in most radiological imaging procedures, such as computed tomography, mammography, dental intraoral, dental panoramic, cone-beam CT, diagnostic fluoroscopy, interventional fluoroscopy, angiography, and radiography.
The tissue weighting factors are for weighting the radiation sensitivity of individual tissues and organs. The greater the probability that radiation is likely to induce fatal cancer in an organ or tissue, the higher the factor. Despite the fact that the wt for the heart is only about 0.0086, the radiation can induce cardiological diseases; therefore, there is a strong limitation of doses to the heart, e.g. in radiotherapy. WT factors summate to 1.
The ED is calculated for a “reference patient”, but the radiation risk per mSv is different depending on gender, age, and population group. For this reason, the ED value is an approximate indicator of possible risk only.
Optimization of radiation protection
In 1997, the European Union introduced the concept of diagnostic reference levels (DRLs), which is a tool for the optimization of radiation protection of patients exposed to ionizing radiation from medical imaging procedures and studies. The establishment and use of DRLs are required in the European directive on Basic Safety standard [5]. DRLS have been established for typical examinations for groups of standard-sized patients or standard phantoms; therefore, the values of DRL quantities should not be used for individual patients. The recommended quantities, symbols, and units for determining reference levels are presented in Table 3. Specifying common units and symbols makes it possible to compare DRLs between different facilities and countries.
The following 4 types of diagnostic reference levels (DRLs) are available [11,22]:
The typical values are obtained from a single healthcare facility or single radiology device and are used to identify a clinical imaging procedure that requires further optimization. It is determined by using the median value of the DLP or CTDIvol distribution.
The local DRLs are obtained from several healthcare facilities in local areas and are used to identify medical devices that require further optimization. It is determined by using the 75th percentile value of the distribution of the appropriate DRL quantity (e.g. 10-20) (Figure 2).
The national DRLs are obtained from multiple healthcare facilities throughout a country and are used nationwide to identify medical devices that require further optimization. The 75th percentile value of the distribution of median values of a DRL quantity at healthcare facilities throughout a country is used as the national DRL.
The regional DRLs are obtained from multiple healthcare facilities throughout several countries within one continent and are used to identify countries within a region without a DRL or for which the national DRL is too high. It is determined by using the median value of the available national values of DRL.
Figure 2
Distribution of total dose length product (DLP) obtained from a few healthcare facilities in the local area. Data distribution from computed tomography (CT) protocol: multiregional CT (chest, abdomen, and pelvis), 3 phases
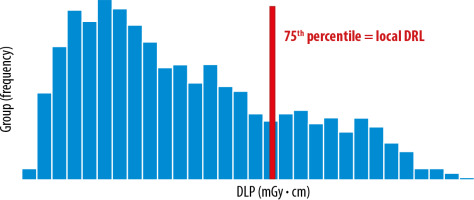
The chart for national DRLs presents the distribution of the median of the total DLP obtained from multiple healthcare facilities throughout a country, and the 75th percentile is determined from this distribution. Similarly, the chart for regional DRLs presents the distribution of the median of the total DLP obtained from multiple healthcare facilities throughout several countries within one continent, and the 75th percentile is determined from this distribution.
The data on DRL quantities from different facilities should refer to procedures that are as similar as possible, with the same clinical task and region of imaging.
The national and regional DRLs should be revised at regular intervals of 3-5 years [11] or more frequently when substantial changes in technology, new imaging protocols, or improved postprocessing of images become available.
Methods for establishing diagnostic reference levels
ICRP survey on how to determine diagnostic reference levels [11]
The latest recommendations for establishing DRL values for different imaging modalities are presented in ICRP Publication 135 – Diagnostic Reference Levels in Medical Imaging. The most important points of the guide are presented below.
DRLs are determined by using the 75th percentile value of the distribution of the appropriate DRL quantity. The ICRP has recommended that DRLs should not be used as alert levels.
Depending on the type of medical imaging, the minimum number of data for determining the amount of the DRL quantity for a specific hospital study varies. As currently agreed, collecting data for at least 20 patients is sufficient to establish a DRL for radiographic examinations. A group of at least 30 patients within the agreed weight range is preferable for CT procedures and diagnostic fluoroscopy, while at least 50 measurements are recommended for mammography. Due to large variability in the procedure complexity the full distribution of dose data is preferable for interventional radiology and cardiology.
The amount of tissue the beam must penetrate has a significant effect on attenuation of the X-ray; therefore, it is important that the analysed data come from studies of patients of similar weight (proposed mean weight about 70 ± 10 kg). However, if dose tracking software is used to collect the data for large numbers of patients, the patient size might be omitted.
To eliminate outliers and data with large errors from the analysis, it is recommended that 5% of the lowest and highest values be excluded from all dose data distribution.
Establishing DRL values for children is more difficult because the variable weight must be included. Within the first 6 months of life, a typical baby’s body weight doubles, and during the first 12 months, it increases 3-fold. The European Guidelines on DRLs for paediatric imaging recommended the creation of DRLs for 5 weight groups: < 5 kg, 5 – < 15 kg, 15 – < 30 kg, 30 – < 50 kg, and 50 – < 80 kg [23].
Additionally, the age group can be used as a parameter for patient grouping and for the purpose of comparison of proposed new weight-based DRLs with earlier values. There are 5 age groups for which it is recommended to create DRLs: < 1 month, 1 month to < 4 years, 4 to < 10 years, 10 to < 14 years, 14 to < 18 years. The exceptions are head examinations, for which recommendations of age groups are different. In this case, 4 age groups are recommended: 0 to < 3 months, 3 months to < 1 year, 1 to < 6 years, and ≥ 6 years. Age is preferred as the grouping parameter for paediatric head examinations [23].
Diagnostic reference levels and clinical indications
The EUCLID project provides up-to-date clinical DRLs and is considered the most important X-ray imaging task in Europe. The project was implemented by the European Society of Radiology (ESR) in 2017-2021.
Until now, DRLs were defined for an anatomical location (A), with a lack of information on the clinical indication (C) and on the procedure (P). According to the EUCLID approach, inclusion of all this information (C+A+P) strengthens the significance of DRLs and provides a stronger tool for optimization and comparisons between centres or countries [24].
The methodology of the EUCLID project was in line with the ICRP assumptions – DRLs are 75% of the median values of each centre involved in this project.
National diagnostic reference levels in Poland
The current NDRLs in Poland are set out in the Minister of Health regulations concerning the conditions of safe usage of ionizing radiation for all types of medical exposure (Dz. U. 2017 poz. 884). These NDRLs are not based on the analysis of doses from Polish hospitals but are adopted (to the Minister of Health regulations) from other countries. Most of these reference levels were launched in 2005 and have not been updated.
NDRLS were adopted as early as 2005 for adult radiography (Table 4), computed tomography (Table 7), and mammography (Table 8). They have not been updated for 17 years, although the national DRLs should be revised at regular intervals of 3-5 years in accordance with the ICRP recommendations.
Table 4
The available Polish national diagnostic reference levels values adopted from other sources in 2005 for radiographic projections [25]
Anatomical region | Projections | DAP* (mGy • cm2) |
---|---|---|
Head | AP or PA | 1100 |
LAT | 1000 | |
Chest | PA | 200 |
LAT | 1000 | |
Thoracic spine | AP | 2200 |
LAT | 3200 | |
Lumbar spine | AP | 3200 |
LAT | 8000 | |
Pelvis | AP | 5000 |
Abdomen | AP or PA | 5000 |
Table 5
The available Polish national diagnostic reference levels values adopted from other sources in 2011 for paediatric radiographic projec- tions [25]
Table 6
The available Polish national diagnostic reference levels values adopted from other sources in 2011 for fluoroscopy and interventional radiology [25]
Table 7
The available Polish national diagnostic reference levels values adopted from other sources in 2005 for computed tomography [25]
Table 8
The available Polish national diagnostic reference levels values adopted from other sources in 2005 for mammography studies [25]
Anatomical region | Projections | ESD (mGy) |
---|---|---|
Breast | CC | 10 |
MLO | 10 |
In 2011, age groups were introduced for paediatric values (Table 5), and DRLs for fluoroscopy and interventional radiology were added (Table 6). Similarly, they have not been updated for a long time (11 years), which is also inconsistent with the ICRP recommendations.
Western European countries (e.g. Austria, Germany) tend to set NDRL values for adult and paediatric X-ray examinations according to their own surveys by analysing data from their own hospitals. In Poland, DRL values are adapted from other sources for adults, and there are no specific values for paediatric fluoroscopy and CT.
Plain radiography
NDRLS were adopted as early as 2005 for adult radiography. They have not been updated for 17 years, although the national DRLs should be revised at regular intervals of 3-5 years in accordance with the ICRP recommendations.
The DRLs are determined for standard radiography projections (AP/PA/LAT) and are expressed as the dose area product. On the list of anatomical regions for which DRLs have been determined, the lower/upper limbs and the cervical spine are missing above (Table 4).
Values of DAP for adult radiography are determined for a standard adult patient – 170 cm tall and weighing 70 kg.
Table 5 presents values of NDRLs for paediatric radio-graphy.
The NDRLs are determined for different age groups and are expressed as the dose area product. On the list of anatomical regions for which paediatric DRLs have been determined, the lower/upper limbs and the whole spine are missing above (Table 5).
Fluoroscopy and interventional radiology
The NDRLs are determined for some interventional procedures and are expressed as the dose area product, for 2 procedures as exposure time additionally. Oesophagogastroduodenoscopy (OGD) and urography are missing above from the listed procedures (Table 6).
Computed tomography
The NDRLs are determined for single anatomical region CT examinations only and do not take into account the clinical indication, e.g. there are no DRL for low-dose examination for chest CT, which could be used for chest cancer screening programs. They are expressed as the dose length product and computed tomography dose index (volume). Table 7 shows that there are no multi-region CT procedures, such as abdomen/pelvis, chest/abdomen/pelvis or neck/chest/abdomen/pelvis, which account for about 60% of all oncological examinations – based on data collected from The Maria Sklodowska-Curie National Research Institute of Oncology in Warsaw.
Mammography
NDRLs are determined for the standard mammographic projections (CC and MLO) and are expressed as the entrance surface dose (Table 8).
Discussion
In recent years, an increasing number of radiological procedures using ionizing radiation have been performed, which forces an even greater focus on radiological protection of the patient [1,2].
Many institutions in the world supervise the radiological protection of patients. Country-specific guidance is provided by the IAEA. In 2014, the IAEA published Radiation Protection and Safety of Radiation Sources: International Basic Safety Standards. The operation of the IAEA is based on the recommendations of the UNSCEAR and the International Commission for Radiological Protection (ICRP). The above recommendations are also based on the European Directive on Basic Safety Standards 2013/59/EURATOM (BSS Directive), which lays down basic safety standards to protect against the dangers of exposure to ionizing radiation [3-6]. There are 3 institutions established in Poland to supervise and inspect activities related to radiation exposure [7]. They are the National Atomic Energy Agency, the State Provincial Sanitary Inspectorate, and the Chief Sanitary Inspectorate. The main document regulating the use of ionizing radiation in all types of activity is the Atomic Law (2019) and the implementing acts to the statute, in particular the Minister of Health regulations concerning the conditions of safe usage of ionizing radiation for all types of medical exposure (Journal of Laws of 2017, item 884).
To date, a lot of dose tracking software (DTS) has appeared on the market, which enables automatic collection of doses and the analysis of large groups of studies, the so-called big data. In addition, they eliminate the risk of making a mistake when manually writing down the doses from each test [8,9]. They also allow alerts to be set up to let users know when the dose has been exceeded for a given study, allowing optimization of test protocols [10]. Unfortunately, most of the DTS are not free (Table 1), but the benefits of implementing this software mean that it might be cost-effective in the case of large heath care providers as well as for small facilities.
The first step in dose optimization is to enter the common units for a given examination modality [11]. This enables doses to be compared across sites, countries, and continents. The doses and common units proposed by the ICRP for determining DRLs are presented in Table 3. Institutions that use other units when determining doses should follow the recommendations and introduce the proposed symbols and units.
The current DRLs for adult and paediatric X-ray exa-minations in many European countries are determined on the basis of the analysis of doses from these countries. But in some countries the DRLs are adapted, which is the situation in Poland, and in some other countries they do not exist at all [22]. The International Commission for Radiological Protection has provided a guide for the determination of DRLs in ICRP Publication 135 – Diagnostic Reference Levels in Medical Imaging. The minimum number of tests necessary to determine DRLs for radio-graphy (20), CT (30), fluoroscopy (30), and mammography (50) has been defined. The next necessary step is therefore to determine local DRLs, then (on their basis) to define national DRLs and establish regional (e.g. Euro-pean) DRLs. DRLs should be determined taking into account sex, age, and weight, as well as not for a standard patient (170 cm tall and weighing 70 kg). For analysis of 100 or more studies, the patient’s weight may be omitted according to ICRP135 [11].
It is important to take into account the clinical indications of the research when determining the DRL. For example, in CT scans, the dose for a low-dose chest screening is different than the dose for a standard chest screening. Attempts have been made to determine DRLs taking into account clinical indications for CT, interventional radiology, and radiography, the results of which were presented in the European Study on Clinical Diagnostic Reference Levels for X-ray Medical Imaging [24].
In Poland, all applicable DRLs are presented in the Minister of Health regulations concerning the conditions of safe usage of ionizing radiation for all types of medical exposure (Dz. U. 2017 poz. 884). Unfortunately, all existing DRLs are adapted from other countries [7,22]. They are not based on the analysis of doses from Polish hospitals. For radiography (Table 4), mammography (Table 8), and CT (Table 7), these levels have been the same for 17 years, while for interventional radiology, fluoroscopy (Table 6), and paediatric radiography (Table 5), they have been the same for 11 years, despite the fact that national DRLs should be revised at regular intervals of 3–5 years, in accordance with the ICRP recommendations [11]. DRLs for dental CBCT do not exist at all, but they do exist for dental intraoral. Therefore, it is necessary to determine local and national DRLs in Poland based on the analysis of doses from recent years and to monitor their changes on an ongoing basis.
Future directions
The current NDRLs in Poland should be updated for all radiological procedures. Due to the increasing number of CT studies, it is worth noting that the DRLs are established only for single anatomical regions for CT examinations (Table 7). However, based on data collected from The Maria Sklodowska-Curie National Research Institute of Oncology in Warsaw, about 60% of all oncological CT examinations are multiregional (chest-abdomen-pelvis and neck-chest-abdomen-pelvis). It is necessary to determine DRL levels for these studies based on data collected from oncology hospitals.