Introduction
Vascular diseases affect the circulatory and lymphatic systems, ranging from pathologies in arteries, veins, and lymph vessels to blood disorders that compromise circulation and threaten limbs and organs [1]. These conditions are the most prevalent in the elderly, with 4.7% of the population aged 60 years and older having at least 2 cardiovascular and metabolic diseases [2]. Additionally, 30% of octogenarians and 20% of nonagenarians have one affected arterial territory. The prevalence of the main pathologies including peripheral arterial disease (PAD), carotid artery atherosclerotic disease, and abdominal aortic aneurysm (AAA) is 3.7%, 3.9%, and 0.9%, respectively [3]. The pathologies involving the venous system also greatly impact the morbidity and mortality of the patients, with an estimated prevalence between 5% and 30% in the adult population. In the year of 2014, the American Venous Forum identified varicose veins in > 30% and > 10% of advanced venous diseases in the National Venous Screening Program in the United States [4].
Commonly used imaging techniques for the diagnosis of vascular diseases involve ultrasonography (USG), computed tomography angiography (CTA), and magnetic resonance angiography (MRA), and they assist in the characterization of the current conditions and in decision-making; nevertheless, they only offer a structural image and evaluate patency and flow, with the limitation in most cases in offering a prognostic value [5].
Recently, new modalities of imaging studies have been developed, like molecular imaging through nuclear medicine, a biomedical discipline that enables the visualization, characterization, and quantification of biological processes at the molecular and cellular levels such as inflammation, proteolysis, angiogenesis, and apoptosis [6,7]. Some of these techniques include the ones that involve computed tomography (CT) – essentially positron emission tomography (PET) and single-photon emission computed tomography (SPECT) [8]. Conversely, the field of magnetic resonance imaging (MRI) has developed nanoprobes for different targets in different pathologies like cancer, atherosclerosis, and obesity [9].
In the present narrative review, we describe the use of molecular imaging in the main entities that affect arterial systems as well as the different techniques and radiotracers that have shown evidence in the diagnosis, follow-up, and prognosis, as well as their specifications, advantages, and limitations.
Aortic aneurysms
An aneurysm is a segmental vessel dilatation exceeding its average diameter by 50%, and it is the most common entity that affects the human aorta. The aortic aneurysm (AA) is in most cases asymptomatic until rupture, with a high mortality of 85 to 90%; thus, it is important for prompt diagnosis, evaluation, and treatment by a vascular surgery team [10]. In this process, several complex pathophysiological mechanisms are involved in the progression of AA, which are potential targets in molecular imaging such as inflammation, angiogenesis, proteolysis, and extracellular matrix remodeling [11,12]. In PET/CT, one of the most commonly used tracers is fluorine-18-fluorodeoxyglucose-6-phosphate (18F-FDG), which accumulates in sites of increased glycolysis such as inflammation [13] and cells with high metabolic activity such as cancer cells and macrophages. Recent literature correlates 18F-FDG uptake with vessel wall pathology (Figures 1A-C, 2A-C) and increased rates of rupture. An important limitation is the poor specificity in inflammatory states, but cells related to this process play crucial roles in AA pathogenesis [14].
Figure 1
18F-FDG PET/CT axial view of an abdominal aortic aneurysm (AAA) in a patient with a pancreatic neuroendocrine tumour (this patient refused treatment by the vascular surgery team in our institute). A) Simple CT of a 7.4 × 7.6 cm AAA (white arrow) with chronic intraluminal thrombus (hollow white arrow). B) Hybrid image showing an increased 18F-FDG uptake at the thrombus periphery (hollow white arrow) and the aortic lumen. C) PET scan increased 18F-FDG uptake at the thrombus periphery (black arrow)
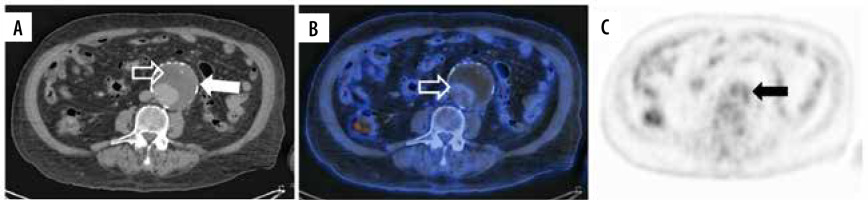
Figure 2
18F-FDG PET/CT coronal projection of an AAA in a patient with IgG4 disease Mikulicz phenotype with a previous endovascular reparation (asterisks) with an aortoiliac graft in 2015. A) Simple CT of AAA with a diameter of 5.9 × 5.6 cm (white arrow). B) Hybrid image shows increased radionuclide uptake in the dilated aortic wall (hollow white arrow). C) PET scan with an increased 18F-FDG uptake (black arrow)
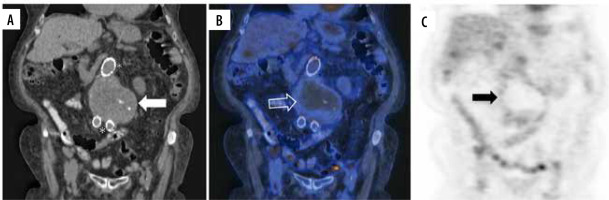
Infective native aortic aneurysm (INAA), first described in 1885 by Osler as “mycotic aneurysm”, is another entity in which 18F-FDG has been used. Recently, the European Society for Vascular Surgery (ESVS) in a Delphi consensus proposed criteria for the diagnosis of this aetiology, as described in Table 1 [15,16]. One of the biomarkers expressed by monocytes/macrophages is the chemokine receptor type 2 (CCR2) that mediates the leukocyte traffic to the arterial wall lesion, which has been analysed in human AA tissue. Copper-64 (64Cu)-1,4,7,10-tetraazacyclododecane-1,4,7,10-tetraacetic acid (DOTA)-extracellular loop 1 inverso (ECL1i) has been developed for targeting CCR2 in murine models, demonstrating its potential to assess aneurysm rupture with an increased PET signal in specimens that present this complication [17]. In another preclinical study, integrin αvβ3 was studied because of its high expression in proliferating endothelial and smooth muscle cells, which leads to neovascularization [18]. Gallium-68 (68Ga)-labelled arginine-glycine-aspartate peptide (RGD) is a radiotracer used for this purpose, which has shown differences in comparison with 18F-FDG using microPET imaging in Wistar rats [19]. Metalloproteinases (MMPs) are another important target involved in extracellular matrix remodeling. In murine models, some of the nucleotides used are RP782, an indium-111(111In)-labelled pan MMPs-tracer that can detect the activation of these enzymes in carotid aneurysms using micro-SPECT/CT, and 64Cu-RYM2 for PET/CT, which has been used in apolipoprotein E-deficient mice with an AA induced by angiotensin II infusion showing a specific aortic signal that correlates with ex-vivo MMPs activity [20,21].
Table 1
ESVS Delphi consensus 2022 criteria for the diagnosis of INAA
These translational studies confirm the viability of using molecular images for the analysis of the primary biological process that affects the expansion of the aorta, and their effectivity should be assessed in humans. A more recent nanoprobe is the ultrasmall superparamagnetic particles of iron oxide (USPIO) for MRI, which also targets inflammation of vascular and lymphatic tissue by macrophages that phagocyte USPIO. Newby et al. performed a prospective multicentre open-label cohort study of 342 patients with a diagnosis of abdominal aortic aneurysm (AAA), who were classified by the presence of USPIO enhancement and were monitored with serial ultrasound and clinical follow-up for 2 years. The results show that USPIO enhancement can predict the growth rate (p = 0.042) and risk of aneurysm rupture or repair (p = 0.308). Also, the authors found a correlation between the USPIO enhancement and current smoking, being an independent predictor of growth. They concluded that USPIO-MRI can be helpful in the decision-making in treatment but requires further investigation [22,23].
Prosthetic aortic graft infection
Aortic graft infection (AGI) can occur when microorganism colonization develops in a primary prosthesis following open surgical reconstruction or an endovascular aortic repair (EVAR) [24]. Although it is a late, rare complication with an incidence ranging from 0.16% to 0.77%, this is a life-threatening condition with a mortality rate of 18-50% [25].
When AGI is suspected, imaging studies should be performed after a physical examination and blood test for the screening of an infection state. CT is the main diagnostic modality, and the signs of infection include local fluid perigraft retention, air bubbles, thickening of graft wall, adjacent blurred fat, and soft tissue swelling. However, these changes are not present in all patients and can occur in other conditions like haematoma, lymph collection, or fibrosis, which makes the differential diagnosis more difficult [26,27].
The radionuclide most widely applied for AGI is 18F-FDG for PET/CT with a sensitivity of 93% and specificity of 70%, which has also shown evidence in imaging for the identification of lethal complications such as fistulas into adjacent structures. Nevertheless, results can show a rate of false-positive cases due to the inflammatory reaction to synthetic grafts, mediated by macrophages and fibroblasts [25-27]. In some studies, technetium-99-labelled white blood cell (99mTc-HMPAO-WBC) scintigraphy with SPECT/CT has shown greater specificity for detecting AGI than FDG for detecting infection and evaluating its extension; for example, de la Rubia-Marcos et al. demonstrated a sensitivity close to 100% in their study of 30 patients with suspect of AGI. However, the small groups of patients in the studies described in the literature do not give solid evidence for the use of scintigraphy [28-30].
Peripheral artery disease
PAD is a progressive atherosclerotic process that results in stenosis or occlusion of non-coronary blood vessels. PAD is a manifestation of systemic atherosclerosis and is associated with significant morbidity and mortality [31,32], which is estimated to affect 202 million people worldwide, with a prevalence of 23.5% [33].
For the screening of patients with PAD, non-invasive tools such as the ankle-brachial index (ABI) and toebrachial index (TBI) are common. Still, these arterial haemodynamic measures can be missing characteristics like medial calcification and incompressible vessels, which are an important differential with diabetes mellitus. Duplex ultrasound, CTA, and MRA can be performed; however, these methods only evaluate arterial patency. The gold standard for diagnosing PAD is catheter-based angiography, which is only recommended for patients undergoing endovascular revascularization because it is an invasive method [34].
Nuclear imaging methods offer new potential approaches to studying the progression of PAD. 18F-FDG PET/CT has shown evidence for the identification of atherosclerotic changes in the vessel wall targeting inflammation by macrophages in metabolic active plaques [5]. Studies in carotid arteries demonstrate that 18F-FDG uptake correlates with remodeling, vessel wall volume, lipid-rich core, fibrous tissue, and the degree of stenosis. Compared to MRI, 18F-FDG uptake also correlates with intra-plaque haemorrhage and the presence of rupture [35]. Calcification also plays an important part in the pathophysiology of PAD, located in the thin fibrous cap overlying the necrotic core of the plaques. 18F-sodium fluoride (18F-NaF) is the most used tracer to visualize calcification activity [36] because NaF binds to hydroxyapatite deposits in the extracellular matrix. In 2010, Derlin et al. described 18F-NaF uptake at vascular wall sites in 75 patients, showing 76% of them with increased uptake, with the highest prevalence in femoral arteries followed by abdominal and thoracic aorta. The study concluded that 18F-NaF is feasible for imaging arterial wall alterations, and that PET/CT could provide information on plaque physiology [37]. Recently, Nogales et al. demonstrated the correlation between 18F-NaF uptake and the histopathological analysis in minipigs, concluding that 18F-NaF accumulates specifically at sites of calcification [38].
Vasculitis
Vasculitis is a heterogeneous chronic disease of unknown aetiology characterized by inflammation in the vessel walls and classified based on the size of the involved vessel [39]. Large vessel vasculitis (LVV) is the most common primary vasculitis [40]. Giant cell arteritis (GCA) and Takayasu arteritis (TA) are the entities that represent the LVV, and both have as a central feature granulomatous arteritis that affects the aorta and its major branches [41,42]. TA onset usually occurs in patients under 50 years old, whereas GCA appears above that age, with a predilection for branches of carotid and vertebral arteries. Because these entities are not related to positive antineutrophil cytoplasmic antibodies (ANCA), LVV lacks an accepted marker of disease activity. Some tools include erythrocyte sedimentation rate (ESR) and CRP, but these are nonspecifically high. Biopsy and histopathological examination are considered the gold standard of diagnosis, but it is an invasive procedure and may not be possible in every patient [43].
18F-FDG is the most studied PET/CT radiotracer for the diagnosis of LVV, with a sensitivity of 76 to 90% for GCA and 70-87% for TA [44]. The key imaging feature is the increased mural tracer uptake that can also help to distinguish between aortitis and periaortitis [45]. Additionally, 18F-FDG uptake is an indicator of active disease, being a potential tool for follow-up (Figure 3A-C)Blockmans et al. describe 35 patients with GCA, who had a temporal artery biopsy and had undergone 18F-FDG PET/CT before treatment, and then they repeated the scan at 3 and 6 months, showing a fall in the 18F-FDG uptake at 3 months compared with the baseline, but ongoing vessel uptake persisted in more than 50% of patients at 6 months with clinical and biochemical remission [46]. Another technique that may have application in the future is 18F-FDG PET/MRI; however, this method needs improved interpretation parameters [43].
Figure 3
18F-FDG PET/CT sagittal projection of a giant cell arteritis cluster 5 patient. A) Simple CT shows supra-aortic trunks, descending, and abdominal aorta. B) Hybrid image shows increased 18F-FDG uptake of the descending aorta (white hollow arrow) and brachiocephalic trunk. C) PET scan shows hypermetabolism of the aorta (black arrow) and supra-aortic trunks (hollow black arrow)
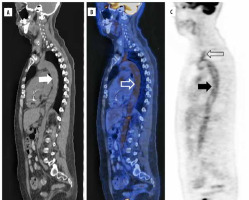
In medium- and small-vessel vasculitis 18F-FDG PET/CT has been used in limited studies, showing increased radiotracer uptake. However, its use has not been fully assessed, and it does not offer additional benefits to the usual screening of these patients [41,47].
Thrombosis and thromboembolic disease in the arterial and venous systems
A variety of nanoprobes have been assessed for the early diagnosis and stratification of the risk of thrombosis in a patient with atherosclerotic disease, especially in coronary arteries. Also, this technique has been employed for imaging thrombi in the jugular vein, aorta, and pulmonary arteries, the latter for evaluating pulmonary thromboembolism [48]. Fibrin-specific low-molecular gadolinium-labelled peptide is the probe for MRI used for this purpose, and the tracers that have been designed include EP-2104R and EP-1873. Previous studies demonstrated increased uptake of contrast at the zone of thrombus formation in coronary arterial disease, intracardiac emboli, and pulmonary emboli in murine models. These preliminary results may be beneficial for the diagnosis and early treatment of the involved pathologies [49,50]. Another important target in thrombosis, besides fibrine, is P-selectin, an adhesion molecule expressed by endothelial cells and platelets on activation. Fucoidan is a type of sulphated polysaccharide derived from brown seaweed that has a high affinity for P-selectin and is used as a radiotracer when technetium-99-labelled (99Tc-fucoidan). Rouzet et al.describe in their study the use of 99Tc-fucoidan for the detection of pathologies associated with P-selectin expression, such as platelets-rich thrombus and transient ischaemic events in animal models, showing low toxicity, no adverse effects, and low cost of the tracer. However, further translational studies are needed for the assessment of the technique [51].
Future directions
Imaging studies are an essential part of diagnosing and treating many pathologies in vascular surgery, although the common image methods like CT and ultrasound are suboptimal for the stratification of patients because they only offer an anatomical image that cannot classify the complication risk and the need for early surgical mana-gement. Despite the availability of information on molecular mechanisms in the pathophysiology of vascular diseases, there remain important gaps in the knowledge of these processes. Some advances in pathogenesis and imaging technology have translated into safer, greater management and less invasive diagnostic and monitoring methods (Figure 4). In the last decade, the application of molecular imaging has not shown enough evidence of diagnostic and prognostic value in vascular pathologies. Some preliminary studies have shown promising results for diagnosis and prognosis. An important limitation, especially in developing countries, could be the cost and availability of these methods. In the oncology area, the use of nuclear imaging has demonstrated effectiveness in decision-making, therapies, and prognosis; the follow-up of patients in a more precise way offers an important advantage in comparison with invasive studies [52]. Also, the radiotracers that are used in the vascular surgery field are relatively accessible. However, well-designed longitudinal studies are needed to obtain reliable information for improving outcomes. If these molecular targets are properly brought to the clinic, it would enhance patient care, from preclinical evaluation to surgical planning and postoperative evaluation.